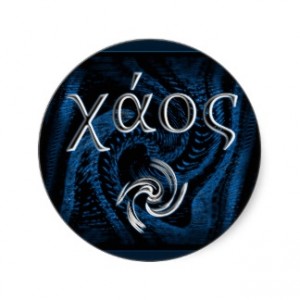
Change, Growth, Decay and Transformation. I learned this from Walter White.
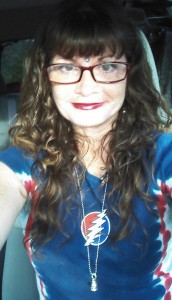
Fermenting beer and wine was done very early in our history.
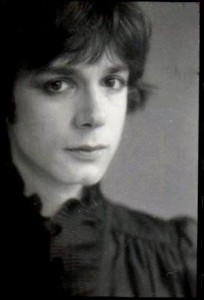
In fact, there is a whole school of historians who think that the beginnings of agriculture lay more in the need to drink beer than in the need to eat food.
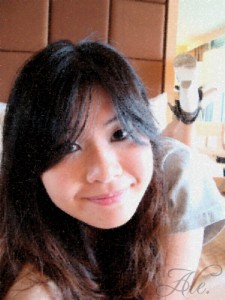
Fermenting is a chemical art as are getting metals from ores, making pottery and glazes, rendering fat into soap, glassmaking, and putting tin and copper together to make bronze.
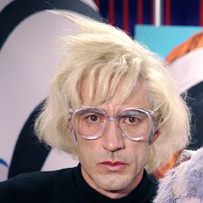
Alchemists who recorded changes and experiments with these processes were the pioneers and precursors of chemistry as we know it today.
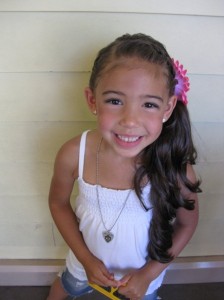
It wasn’t until the seventeenth, maybe even eighteenth century that a clear difference was established between alchemy and chemistry
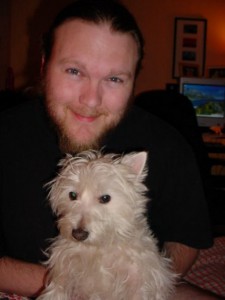
The first metals used by humans were those which could be found on the ground in their natural state, such as gold, silver, copper, tin and the iron that came from the sky in the form of meteorites.
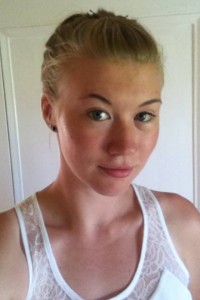
People have found natural gold in Spanish caves dating from the Paleolithic (40,000 BCE).
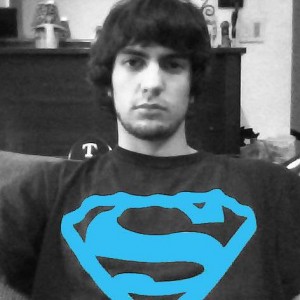
Egyptians made weapons from meteoric iron and they called them “Daggers from Heaven.”
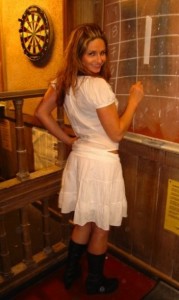
Chemistry is change and nothing effects change more dramatically than fire.
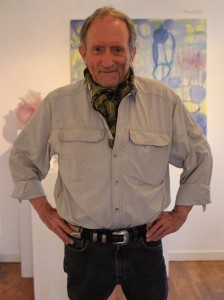
To see water boil, or wood transformed into black charcoal, to see sand turn to glass or metals melt… these must have seemed like magical processes at first and indeed they still seem magical.
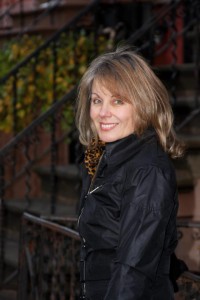
Tin, copper and lead can be taken out of rock merely by heating the rock and this began to be done around 5,000, 6,000 BCE in Serbia (Majdanpek, Yarmovac, Plocnik).
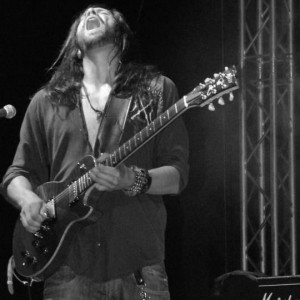
At the Belovode site in Serbia, people seem to have done the first smelting of, for example, a copper axe head (5,500 BCE) from the Vin?a culture.
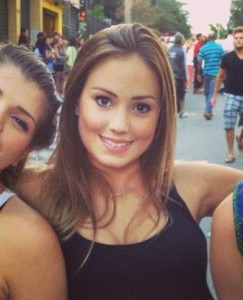
Archaeologists have found early metals from the third millennium BCE in Portugal, Spain and England (Stonehenge).
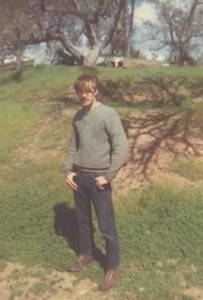
The making of perfume from plants, colors from plants and rocks, these are chemical operations.
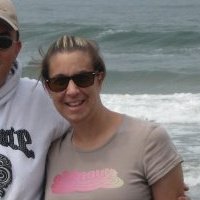
Arsenic is brown, copper can be an intense, beautiful blue or an equally attractive green.
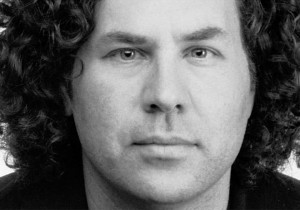
Tin can be silvery gray and iron is red brown as we see so often in the earth around us.
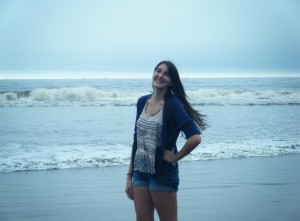
People began to adorn themselves with these colors very early on.
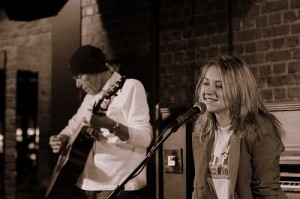
When it was discovered that copper and tin could be put together into a new better metal, a lot of things changed and this major change was called the Bronze Age (3,500 BCE).
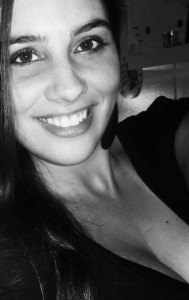
Arsenic was an impurity that occurred in the smelting of bronze.
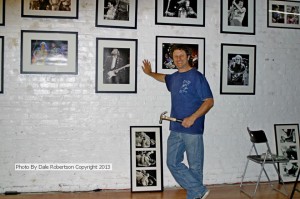
Iron was much more difficult to take out of its native ore than were gold, copper and tin.
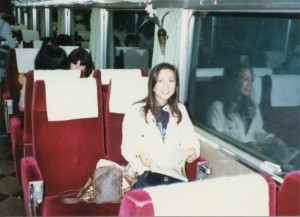
There are substantive claims made for early, very early African iron making, but the traditional account is that Hittites began to work iron in 1,200 BCE and so began the Iron Age.
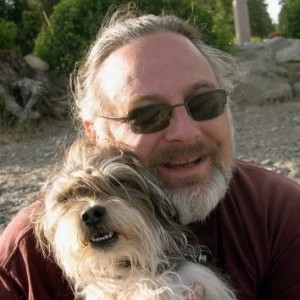
The Philistines who lived along the eastern Mediterranean coast and who gave their name to Palestine became a successful people because they learned to extract and work iron.
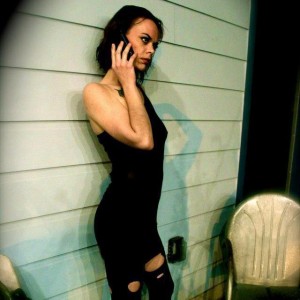
Iron Age metalworking (ferrous metallurgy) began to be done almost worldwide in such places as the Middle East, Near East, Far East, Iran, Egypt, Nubia (Sudan), Anatolia (Turkey), Carthage, Greece, Italy, United Kingdom, China, Japan. Of course, nowadays metal fabrication is done all over the world with sophisticated techniques using argon welding gas and a variety of tools to create some impressive metalwork.
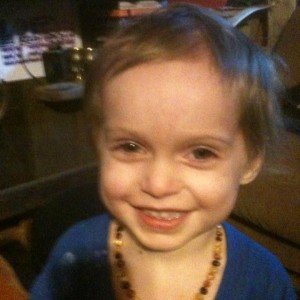
As I have mentioned before, the Chinese invented the blast furnace, cast iron, water powered trip hammers and double acting piston bellows.
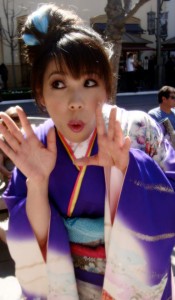
How do these metals exist in different forms and how do they change into other forms was a question that thoughtful people asked very early. These questions are the foundations of alchemy and chemistry.
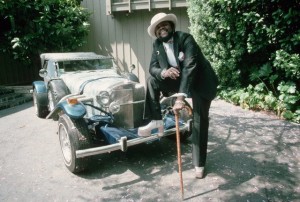
What were the simplest, most fundamental elements?
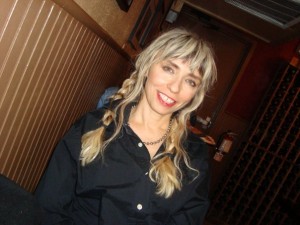
Air, water, earth and fire seemed to be very basic, and then gold, silver, copper, tin.
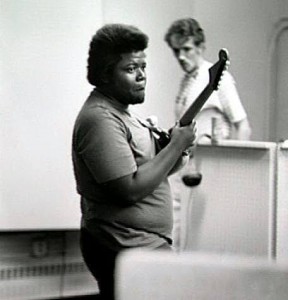
There were even early philosphers who posited an atomic basis for everything.
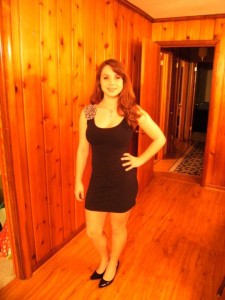
How did they do this?
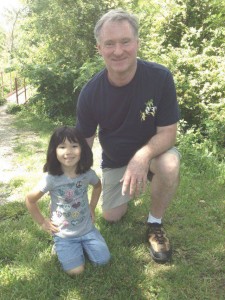
Did they intuit the presence of atoms?
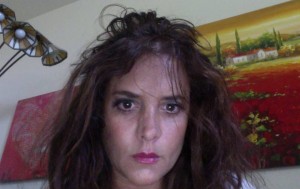
Democritus and Leucippus in Greece and Kanada in India (in the Vaisheshika sutras) created a theory of atomism that wasn’t heard of again until John Dalton began postulating a similar idea in the eighteenth century of our time.
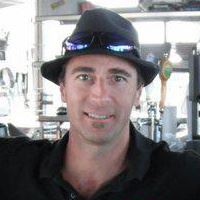
Where were the proofs for such an idea as atomism?
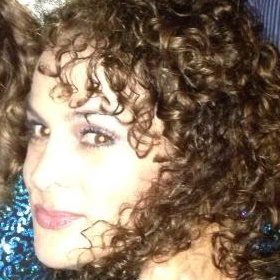
The Greeks in their philosophy and Kanada in his sutras talked about atoms, but there was no real clear evidence of atoms until the twentieth century.
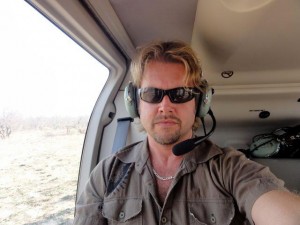
This didn’t stop Epicurus in 300 BCE from claiming that there was a universe of tiny, indivisible parts (atoms = a tomos = un cut able).
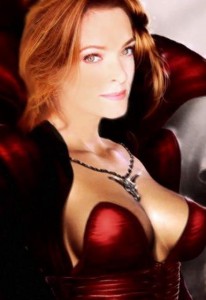
Where was the empirical evidence for this?
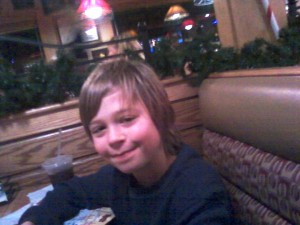
Aristotle, just to name one thinker, denied the existence of atoms completely, and Hippocrates thought and said that the human body was composed of four humors, an idea that lasted well into modern times, almost to the Age of Enlightenment.
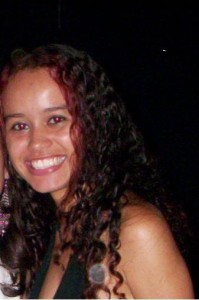
The four humors were blood, fire, earth and phlegm, and these created the termperaments.
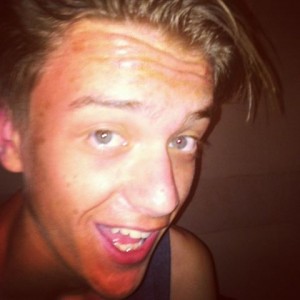
Blood made for a sanguine temperament or mood.
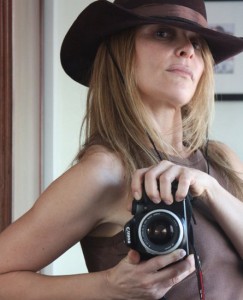
Fire was choleric.
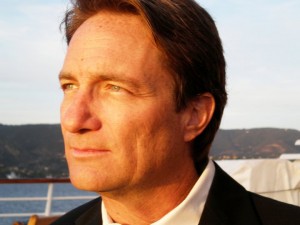
Water was phlegmatic and earth was melancholic.
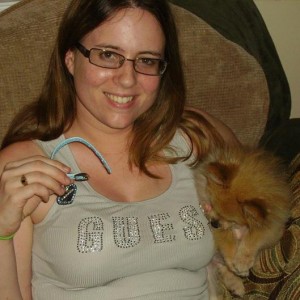
It was quite an elaborate system and it held sway up into the eighteenth century of our time.
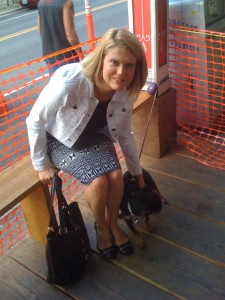
We still use these terms, of course, but don’t believe in them literally.
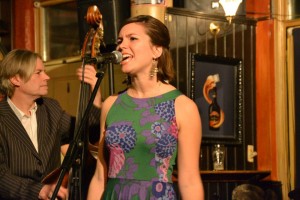
Sentences such as, She had a sanguine disposition.
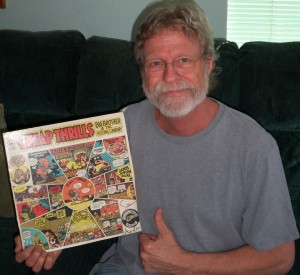
He had a choleric nature.
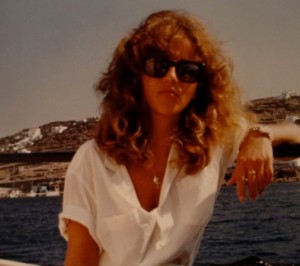
So and so was so phlegmatic and in a melancholy mood that day.
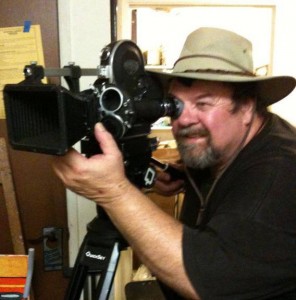
Epicurus, on the other hand, not only said that we live in a world of atoms, but that it is incumbent upon us to lead balanced, harmonious lives.
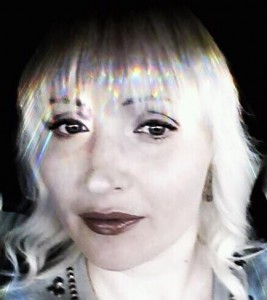
How he went from one of these ideas to the other is very Greek, but it is not at all “epicurean” as we use the word today.
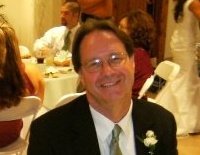
Quite the contrary, in fact.
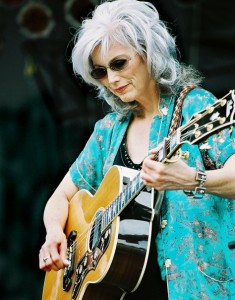
Lucretius sought to explain the thinking of Epicurus to a Roman audience and so he wrote De Rerum Natura (On the Nature of Things) in 50 BCE, where he explains the idea of atomism, what the mind and soul are, sensations, thought, the development of the physical world and many heavenly phenomena.
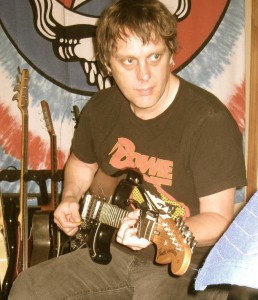
The self confidence of these early thinkers is staggering.
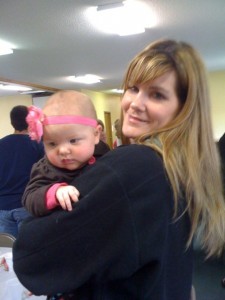
They were erecting whole worlds out of thin air.
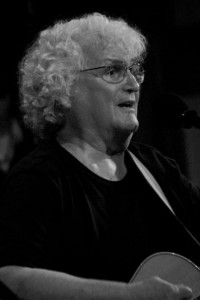
They would never see an atom.
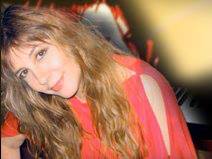
No one would for a long time, and yet they stated unequivocally that atoms were there and were the basis for everything.
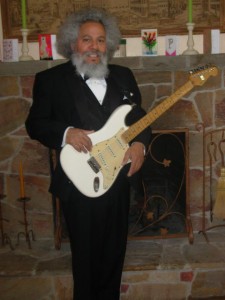
Pliny the Elder took a more practical, concrete approach to all of this and described with accuracy many minerals and properties of earth.
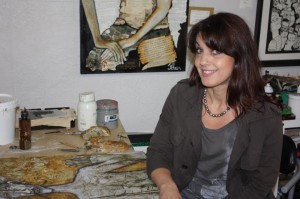
A Persian who wrote in Arabic, Jabir ibn Hayyan studied Aristotle’s idea of air, earth, fire and water in addition to two philosophical elements: sulphur (combustability) and mercury (the metallic properties) and thus developed the elemental system used in medieval alchemy.
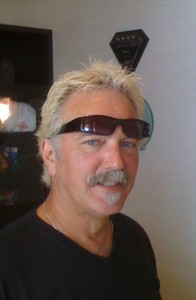
The three metallic principles: sulphur to flammability or combustion, mercury to volatility and stability, and salt to solidity became the tria prima of the Swiss alchemist Paracelsus who reasoned that Aristotle’s four element theory appeared in bodies as three principles.
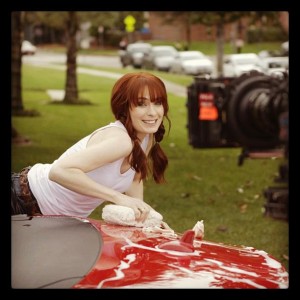
Paracelsus saw these principles as fundamental and justified them by recourse to the description of how wood burns in fire.
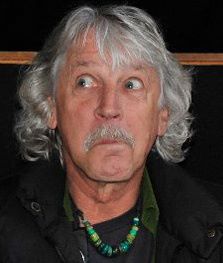
Mercury was the cohesive principle, so that when it left in smoke the wood fell apart.
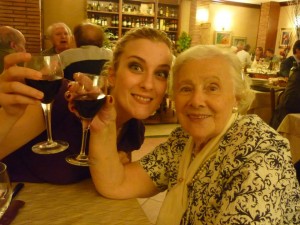
Smoke described the volatility (the mercurial principle), the heat-giving flames described flammability (sulphur), and the remnant ash described solidity (salt).
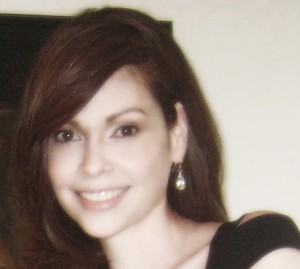
Alchemy is defined by the Hermetic quest for the philosopher’s stone, the study of which is steeped in symbolic mysticism, and which differs greatly from modern science.
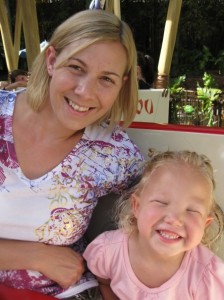
Alchemists wanted to make transformations on an esoteric (spiritual) and/or exoteric (practical) level.
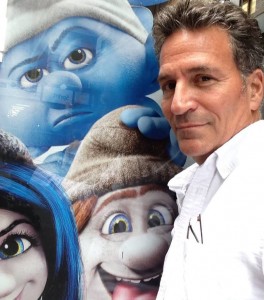
It was the exoteric aspects of alchemy that contributed heavily to the evolution of chemistry in Greco-Roman (Hellenistic) Egypt, in the Islamic golden age, and then in Europe.
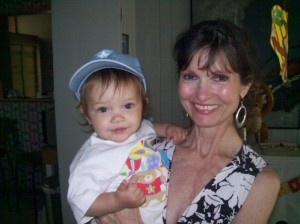
Alchemy and chemistry share an interest in the composition and properties of matter, and prior to the eighteenth century were not separated into distinct disciplines.
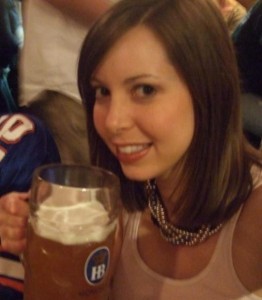
The term chymistry has been used to describe the blend of alchemy and chemistry that existed before this time.
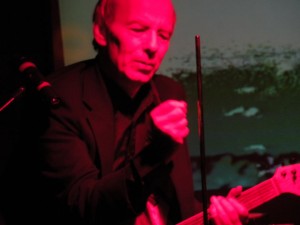
The earliest Western alchemists, who lived in the first centuries of the common era, invented chemical apparatus.
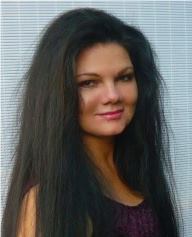
The bain-marie, or water bath is named for Mary the Jewess, whose work gives the first descriptions of the tribikos and kerotakis, types of stills.
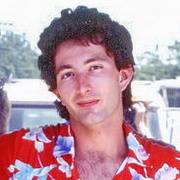
Cleopatra the alchemist described furnaces and has been credited with the invention of the alembic, although there are several claimants for this title.
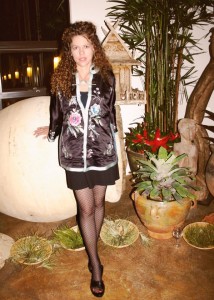
Jabir ibn Hayyan set the foundations for the experiments and their methodology which influenced alchemists in the Islamic, and, thus, later the European world in the twelfth century.
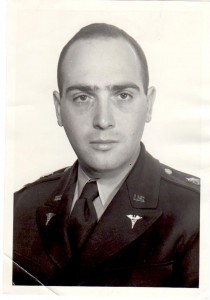
In the Renaissance, exoteric alchemy remained popular in the form of Paracelsian iatrochemistry (iatros = doctor, physician) while spiritual alchemy flourished in its Platonic, Hermetic, and Gnostic roots.
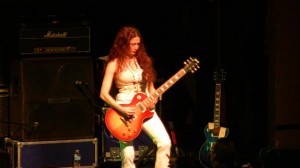
The quest for the philosopher’s stone, a legendary substance, allegedly capable of turning inexpensive metals into gold, was not outmoded by scientific advances, but was still the domain of respected scientists and doctors until the early eighteenth century.
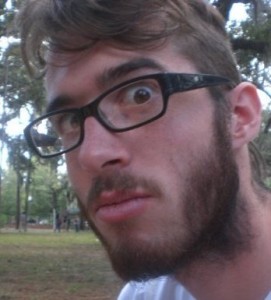
Jan Baptist van Helmont, Robert Boyle and Isaac Newton were all alchemists as well as chemists.
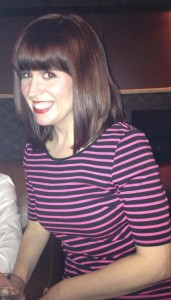
They still were searching for a formula that would transform base metals into gold, although Newton warned one colleague about advertising that fact.
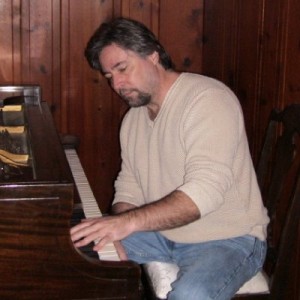
The thing about alchemy was that there was no orderly, logical system for naming new compounds and the alchemical language was codified, secretive, esoteric and vague.
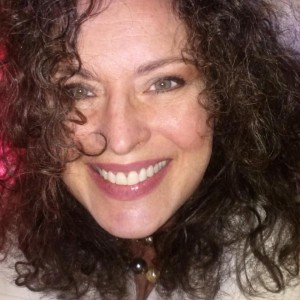
Different terms meant different things to different people.
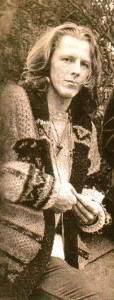
Science demands openness and complete honesty.
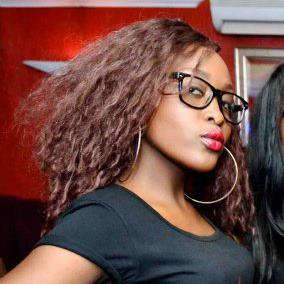
There is no place in it for concealment and protection of sources.
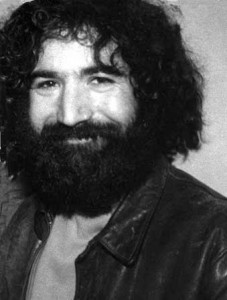
From The Fontana History of Chemistry (Brock, 1992):
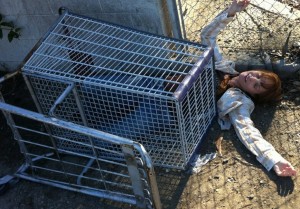
The language of alchemy soon developed an arcane and secretive technical vocabulary designed to conceal information from the uninitiated. To a large degree, this language is incomprehensible to us today, though it is apparent that readers of Geoffrey Chaucer’s Canon’s Yeoman’s Tale or audiences for Ben Jonson’s The Alchemist understood the alchemical language in these narratives well enough to laugh at it.
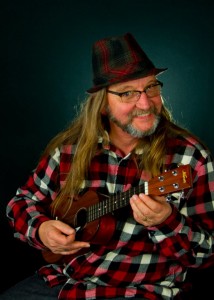
Chaucer’s tale exposed the more unethical, hypocritical, thieving side of alchemy, especially the manufacture of counterfeit gold from cheap substances.
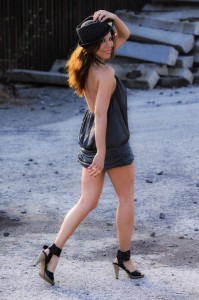
Dante Alighieri banished all alchemists to the Inferno.
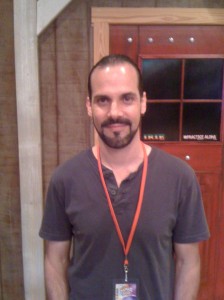
In 1317, the Avignon Pope John XXII ordered all alchemists to leave France because they were counterfeiting money.
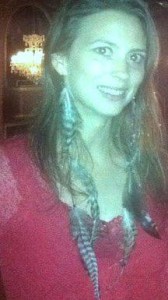
A law was passed in England in 1403 which made the “multiplication of metals” punishable by death.
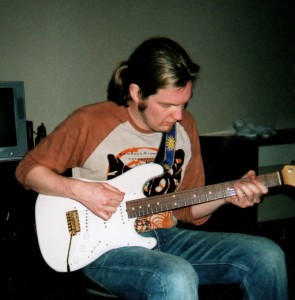
Yet royalty and privileged classes still sought to discover the philosopher’s stone and the elixir of life for themselves.
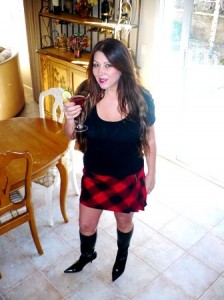
Illusions do not die easily.
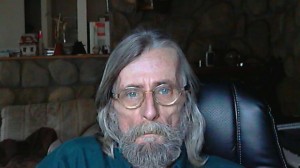
Potent is the lure of free money, as we still see today.
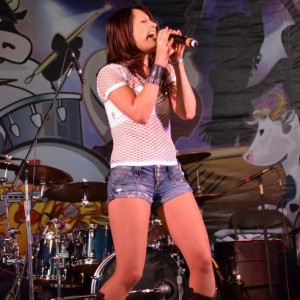
The goal of legitimate scientific inquiry was to make experiments reproducible, but one of the major aims of alchemists was to hide their methods, so there was a basic conflict here
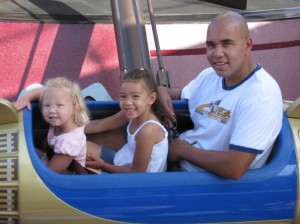
There was a need for an honest scientific method where experiments could be repeated by others results reported in a clear language that laid out both what was known and unknown.
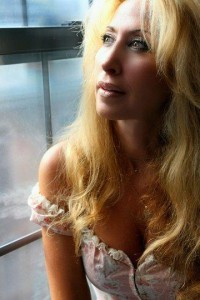
In the Islamic World, Muslims and Arabic speaking Persians were translating the works of the ancient Greeks and Egypticans they were experimenting with scientific ideas.
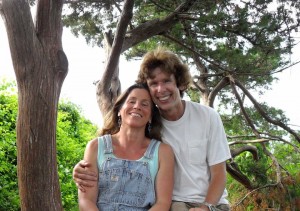
An early scientific method for chemistry began to emerge with the work of the 9th century chemist Jabir ibn Hayyan (known as “Geber” in Europe), who is considered as “the father of chemistry,” just as Antoine Lavolisier was centuries later.
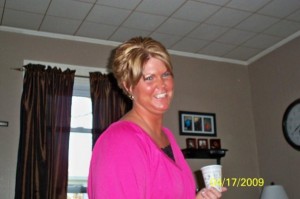
Jabir ibn Hayyan introduced a systematic and experimental approach to scientific research based in the laboratory, in contrast to the ancient Greek and Egyptian alchemists who took a more “magical” approach to their discoveries and findings.
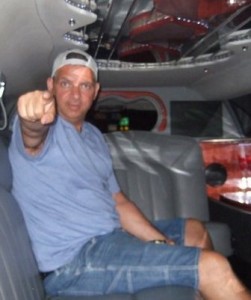
Hayyan invented and named the alembic (al-anbiq), chemically analyzed many chemical substances, composed lapidaries, distinguished between alkalis and acids, and manufactured hundreds of drugs.
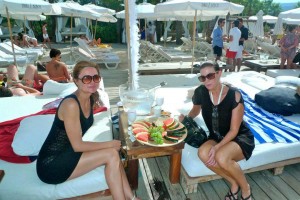
Jabir ibn Hayyan proceeded systematically, refining the theory of five classical elements into the theory of seven alchemical elements and identifying mercury and sulfur as chemical elements.
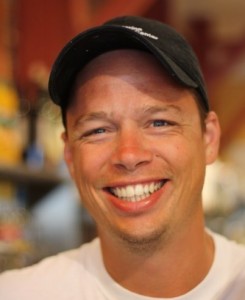
Many chemists in the Persian Arabic world detected early the speciousness of alchemy, particularly the “transmutation of metals” aspect.
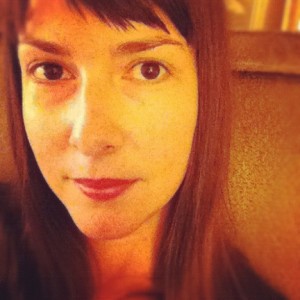
Such heroes of chemistry as Abu al-Rayhan al-Buruni, Avicenna (to use his European name), Al-kindi and al-Tusi who wrote about the conservation of mass, noting that a body of matter can, yes, change, but not disappear.
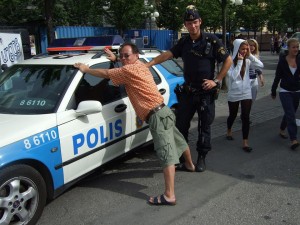
Or appear for that matter, appear out of nowhere.
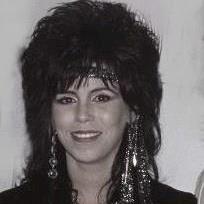
Rhazes (????? ???? ?????? ???? Ab? Bakr Mu?ammad-e Zakariy?-ye R?z?) shined the bright light of reason on the Aristotle Hippocrates theory of the four humors and said, in effect, “Oh, come on, you can’t be serious.”
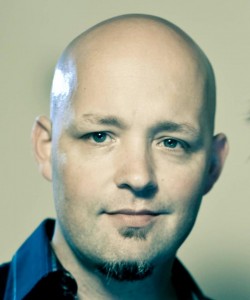
Rhazes went on to design and describe many chemical instruments which are still in use today, the crucible or retort, the alembic and different kinds of chemical stoves.
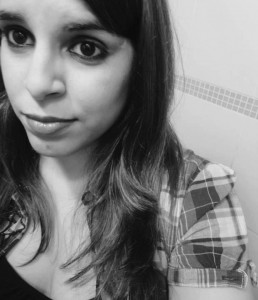
Paracelsus (1493–1541), a Swiss alchemist, also rejected the four humors theory and formed a hybrid of alchemy and science (iatrochemistry), where chemicals, whether made in the laboratory or found in plants, were used for healing.
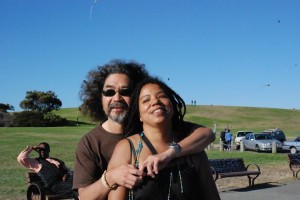
Iatrós ( ?????? “healer”) is Greek for doctor. It is present in such words as pediatrics, psychiatrist, podiatrist.
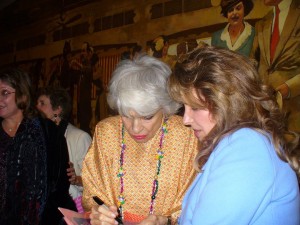
Paracelsus was not perfect in making his experiments truly scientific.
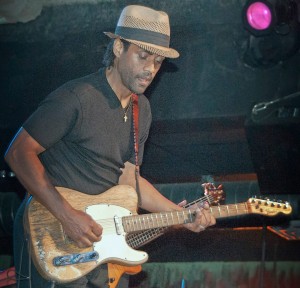
For example, as an extension of his theory that new compounds could be made by combining mercury with sulfur, he once made what he thought was “oil of sulfur”.
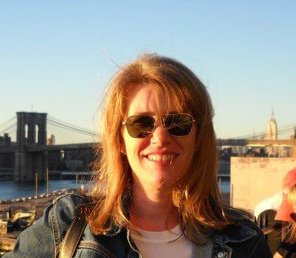
This was actually dimethyl ether which contained neither mercury nor sulfur.
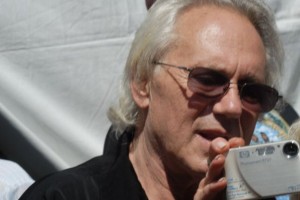
Georg Agricola (1494–1555), who published his great work De Re Metallica in 1556, wanted to improve the refining of ores and their extraction to smelt metals
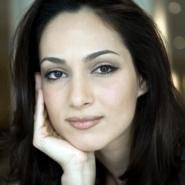
Agricola’s work describes the highly developed and complex processes of mining metal ores, metal extraction and metallurgy of the time.
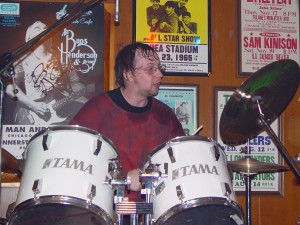
Agricola created a practical base upon which others could build by removing the alchemical mysticism from the proceedings.
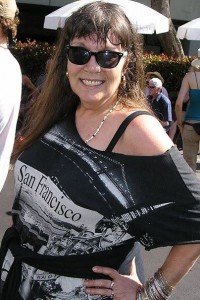
De Re Metallica describes the many kinds of furnace used to smelt ore, and the book stimulated interest in minerals and their composition.
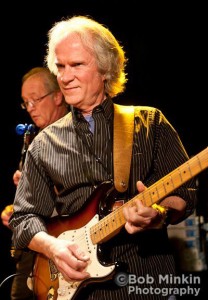
Agricola makes numerous references to the earlier author, Pliny the Elder.
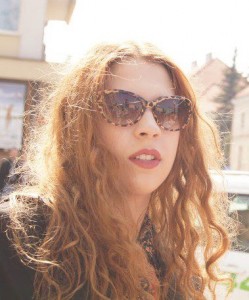
In 1605, Sir Francis Bacon published The Proficience and Advancement of Learning, which is the first clear description of the scientific method.
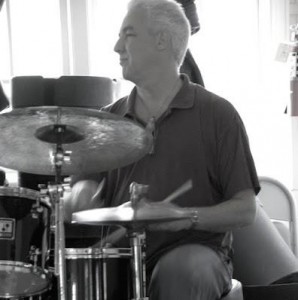
In 1605, Michal Sedziwój published the alchemical treatise A New Light of Alchemy which proposed the existence of oxygen.
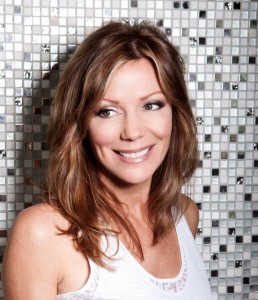
And in 1615 Jean Beguin published the Tyrocinium Chymicum, an early chemistry textbook,containing the first-ever chemical equation.
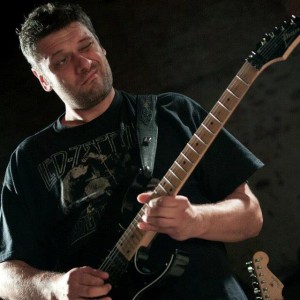
René Descartes published Discours de la Méthode (1637), which also outlines the scientific method.
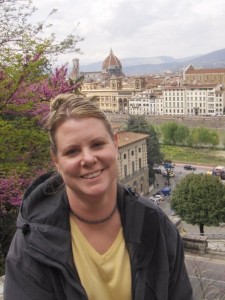
The Dutch chemist Jan Baptist van Helmont’s Ortus medicinae is cited by some as a major transitional work between alchemy and chemistry, and it had an important influence on Robert Boyle.
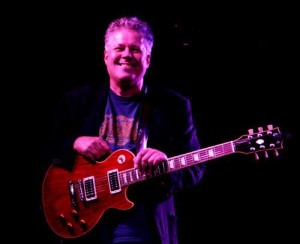
There are numerous experiments in the book which established an early version of the law of conservation of mass.
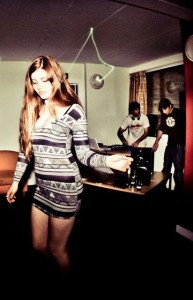
Jan Baptist van Helmont, during the time just after Paracelsus and iatrochemistry, suggested that there are insubstantial substances other than air and coined a name for them, “gas” from the Greek word chaos, so think about that the next time you’re running on empty.
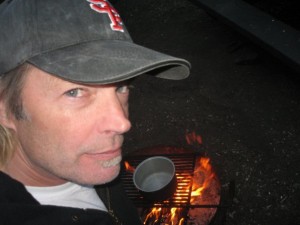
Van Helmont conducted several experiments involving gases.
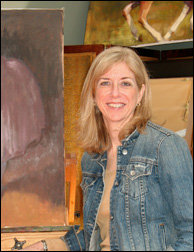
He is also remembered today largely for his ideas on spontaneous generation and his 5-year tree experiment, as well as being considered the founder of pneumatic chemistry.
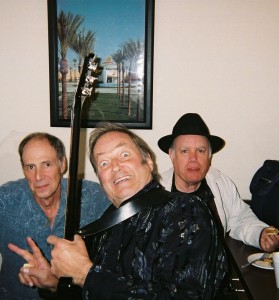
English chemist Robert Boyle (1627–1691) refined the modern scientific method for alchemy and separated chemistry further from alchemy.
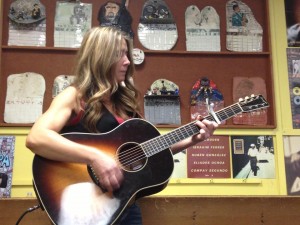
Boyle is regarded today as the first modern chemist, and one of the founders of modern chemistry, a pioneer of the experimental scientific method.
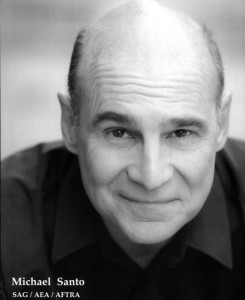
He did not actually discover Boyle’s Law, but he presented and formalized it in 1662.
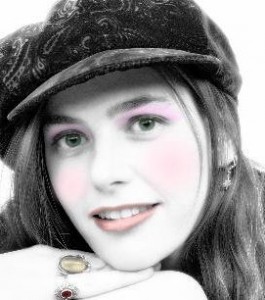
Boyle’s law describes the inversely proportional relationship between the absolute pressure and volume of a gas, given a constant temperature within a closed system.
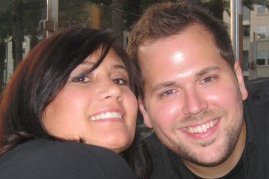
Boyle wrote The Sceptical Chymist in 1661, a cornerstone book in chemistry.
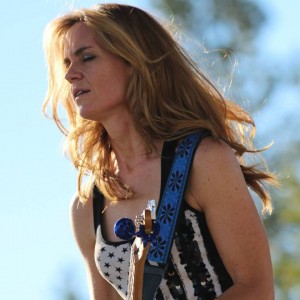
In The Sceptical Chymist Boyle posits that every phenomenon is the result of collisions of particles in motion.
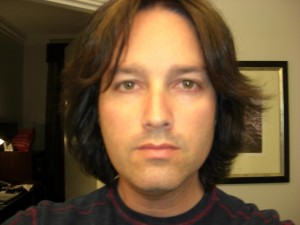
Boyle asks for experimentation and he asserts that experiments show that the classic four humors or elements: earth, fire, air, and water are not enough to explain nature.
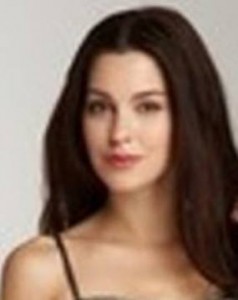
Boyle also pleads that chemistry cease to be subservient to medicine or to alchemy.
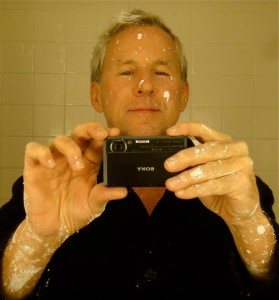
He is really pushing for a rigorous approach to scientific experimentation and he believed that all theories must be proved experimentally before being regarded as true.
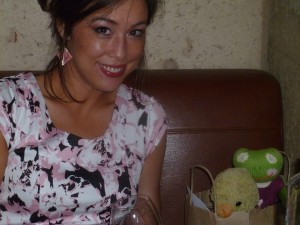
The Sceptical Chymist contains some of the earliest modern ideas of atoms, molecules, and chemical reactions, and marks the beginning of the history of modern chemistry.
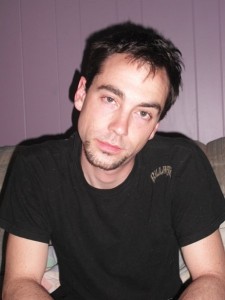
Boyle aimed for that classic scientific goal, reproducible results, and he needed purer chemicals for that.
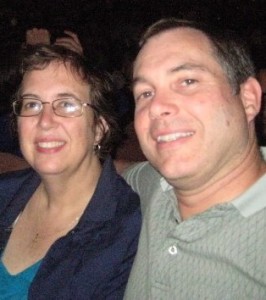
He agreed with René Descartes in explaining and quantifying the physical properties and interactions of material substances.
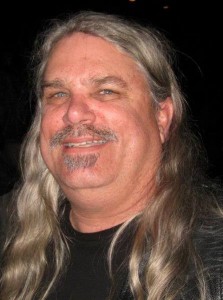
Boyle was an atomist, but he preferred the term corpuscle over atoms, so that would make him a corpuscleist, which sounds a bit silly now.
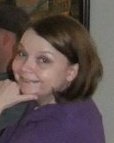
“Atom” merely means uncuttable, and I suppose “corpuscle” would mean a little bodylike thing.
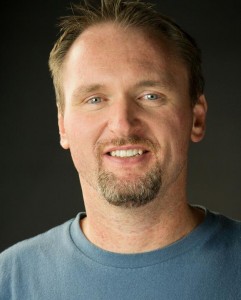
One thing is for sure.
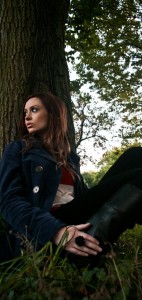
The atom is very cuttable, so the name atom is not very descriptive now.
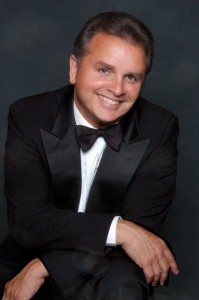
The atom has been split so many times now that even its parts have been split many times and there is no end in sight.
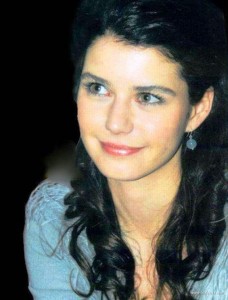
What is the name of the latest found particle of the atom? Found only within the last year? The Higgs Boson, is that it?
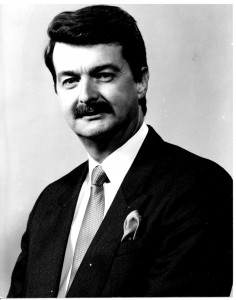
There’s a whole universe inside an atom, just as those science fiction writers in the 1950s promised us.
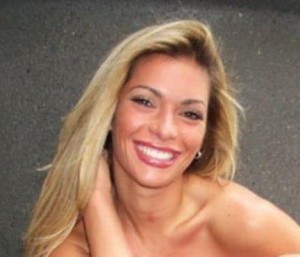
So Boyle thought that the most elemental level of matter was the corpuscle.
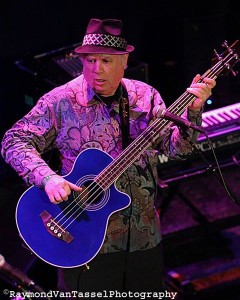
He performed numerous investigations with an air pump and noted that the mercury fell as air was pumped out.
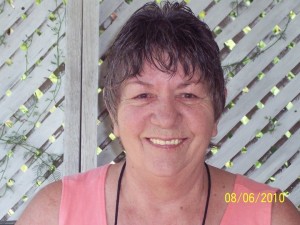
He also observed that pumping the air out of a container would extinguish a flame and kill small animals placed inside, and well as causing the level of a barometer to drop.
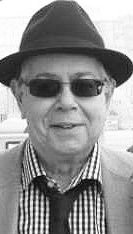
Boyle was in the vanguard of the chemical revolution with his mechanical corpuscular philosophy.
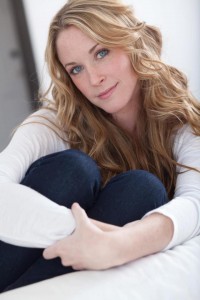
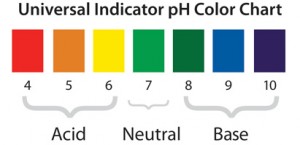
He found time to repeat the tree experiment of van Helmont, and was the first to use indicators, those little slips of paper, which changed colors with acidity.
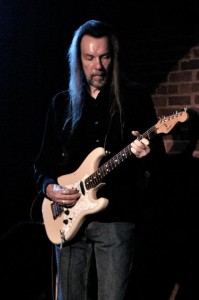
Here is van Helmont’s tree experiment in van Helmont’s own words:
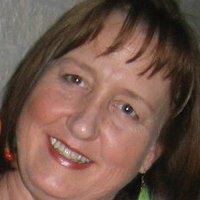
I took an earthen pot and in it placed 200 pounds of earth which had been dried out in
an oven. This I moistened with rain water, and in it planted a shoot of willow which
weighed five pounds. When five years had passed the tree which grew from it weighed
169 pounds and about three ounces. The earthen pot was wetted whenever it was
necessary with rain or distilled water only. It was very large, and was sunk in the ground,
and had a tin plated iron lid with many holes punched in it, which covered the edge of
the pot to keep air-borne dust from mixing with the earth. I did not keep track of the
weight of the leaves which fell in each of the four autumns. Finally, I dried out the earth
in the pot once more, and found the same 200 pounds, less about 2 ounces. Thus, 164
pounds of wood, bark, and roots had arisen from water alone.”
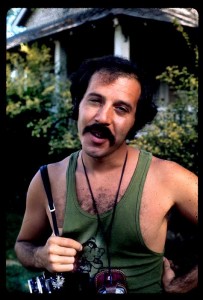
So, really? 164 pounds of wood, bark and roots had arisen from 2 ounces of water alone? What is the main igredient, truly the principal ingredient that van Helmont is omitting here? Could it be… solar power?
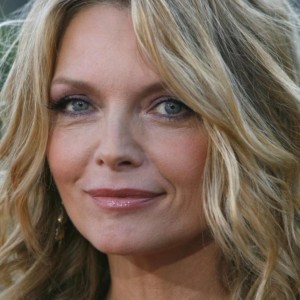
Is van Helmont forgetting anything else?
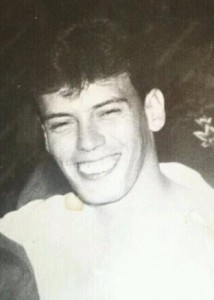
It’s an interesting experiment, isn’t it?
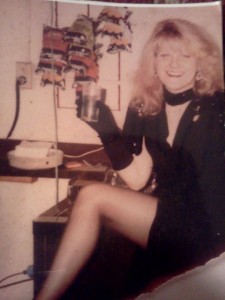
In 1702, German chemist Georg Stahl coined the name “phlogiston” for the substance believed to be released in the process of burning, and thereby set off a couple of centuries of chemical mischief.
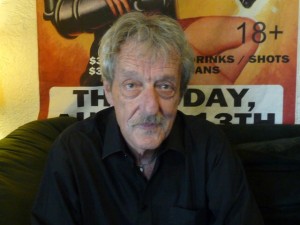
The phlogiston theory postulated a fire-like element called phlogiston, contained within combustible bodies, that is released during combustiuon.
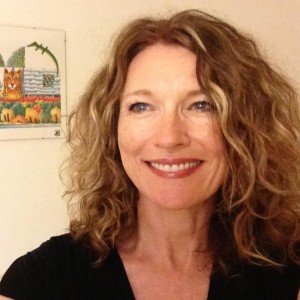
The name comes from the Greek ????????? phlogistón (burning up), from ???? phlóx (flame).
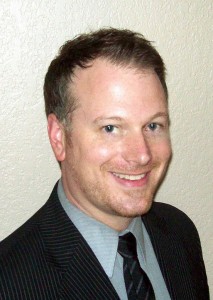
The phlogiston theory was first stated in 1667 by Johann Joachim Becher.
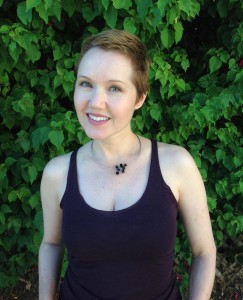
The theory attempted to explain burning processes such as combustion and rusting which are now collectively known as oxidation.
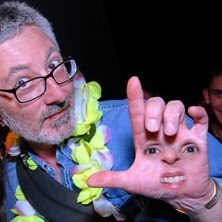
When you buy foods that are rich in anti-oxidants you are trying to keep your insides from rusting and burning, aren’t you?
In general, substances that burned in air were said to be rich in phlogiston; the fact that combustion soon ceased in an enclosed space was taken as clear-cut evidence that air had the capacity to absorb only a finite amount of phlogiston. When air had become completely phlogisticated it would no longer serve to support combustion of any material, nor would a metal heated in it yield a calx; nor could phlogisticated air support life, for the role of air in respiration was to remove the phlogiston from the body.
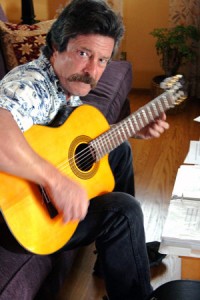
Thus, Becher described phlogiston as a process that was basically the opposite of the role of oxygen in combustion.
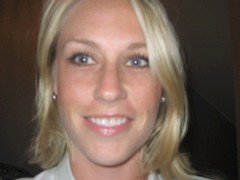
Daniel Rutherford discovered nitrogen in 1772 and used the phlogiston theory to explain his results.
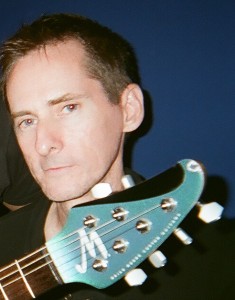
The residue of air left after burning, in fact a mixture of nitrogen and carbon dioxide, was sometimes referred to as phlogisticated air, having taken up all of the phlogiston.
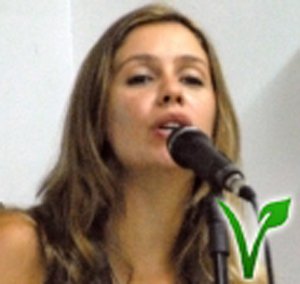
Conversely, when oxygen was first discovered, it was thought to be dephlogisticated air, capable of combining with more phlogiston and thus supporting combustion for longer than ordinary air.
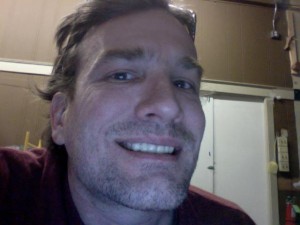
Amazing how an airy nothing of a theory can be so catastrophical to common sense. People believed in this absraction for a long time. They also believed in “ether.” Many serious scientists staked their reputations on the existence of phlogiston and ether.
Around 1735, Swedish chemist Georg Brandt analyzed a dark blue pigment found in copper ore, and demonstrated that the pigment contained a new element, later named cobalt.
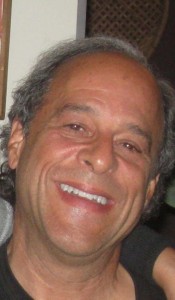
In 1751, a Swedish chemist and pupil of Stahl’s named Axel Fredrik Cronstedt identified an impurity in copper ore as a separate metallic element, which he named nickel.
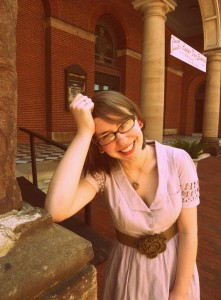
Cronstedt is one of the founders of modern mineralogy.
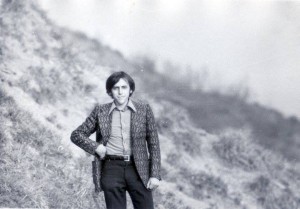
Cronstedt also discovered the mineral scheelite in 1751, which he named tungsten, meaning “heavy stone” in Swedish.
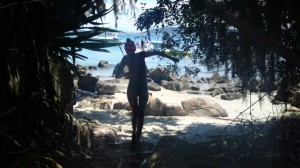
In 1754, Scottish chemist Joseph Black isolated carbon dioxide which he called “fixed air”.
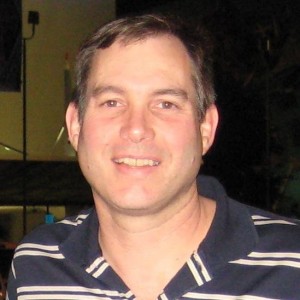
In 1757, Louis Claude Cadet de Gassicourt, while investigating arsenic compounds, created Cadet’s fuming liquid, later discovered to be cacodyl oxide, considered to be the first synthetic organomettalic compound.
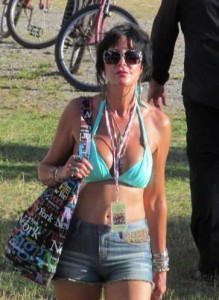
In 1758, Joseph Black formulated the concept of latent heat to explain the thermochemistry of phase changes.
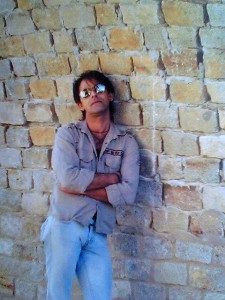
In 1766, English chemist Henry Cavendish isolated hydrogen which he called “inflammable air”.
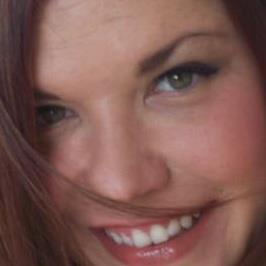
Cavendish discovered hydrogen as a colorless, odourless gas that burns and can form an explosive mixture with air, and published a paper on the production of water by burning inflammable air (that is, hydrogen) in dephlogisticated air (now known to be oxygen), the latter a constituent of atmospheric air (according to the phlogiston theory).
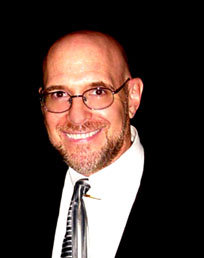
In 1773, Swedish chemist Carl Wilhelm Scheele discovered oxygen, which he called “fire air”, but did not immediately publish his findings.
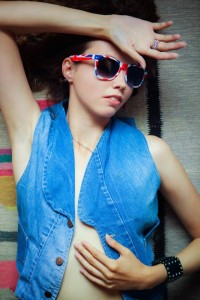
In 1774, English chemist Joseph Priestly independently isolated oxygen in its gaseous state, calling it “dephlogisticated air”, and published his work before Scheele.
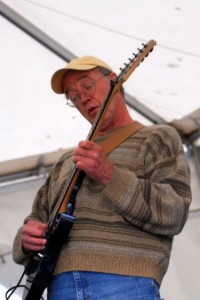
During his lifetime, Priestley’s considerable scientific reputation rested on his invention of soda water, his writings on electricity, and his discovery of several “airs” (gases), the most famous being what Priestley dubbed “dephlogisticated air” (oxygen).
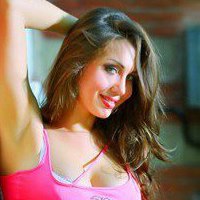
However, Priestley’s determination to defend phlogiston theory and to reject what would become the chemical revoution eventually left him isolated within the scientific community.
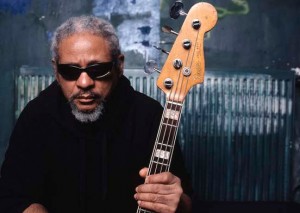
In 1781, Carl Wilhelm Scheele discovered that a new acid, tungsten acid could be made from Cronstedt’s scheelite (at the time named tungsten).
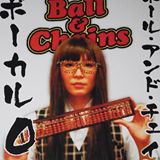
Scheele and Torbern Bergman suggested that it might be possible to obtain a new metal by reducing this acid.
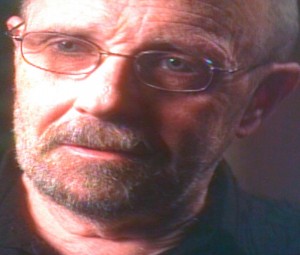
In 1783, José and Fausto Elhuyar found an acid made from wolframite that was identical to tungstic acid.
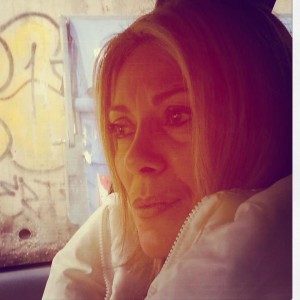
Later that year, in Spain, the brothers succeeded in isolating the metal now known as tungsten by reduction of this acid with charcoal, and they are credited with the discovery of the element.
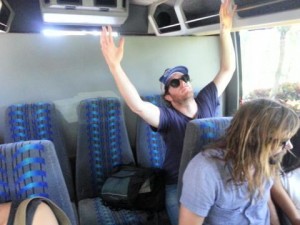
Oliver Sacks wrote an entire entertaining book Uncle Tungsten (Memories of a Chemical Boyhood) about his family and about this metal.
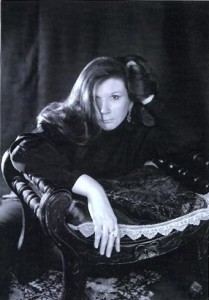
Antoine-Laurent de Lavoisier is celebrated as the father of modern chemistry.
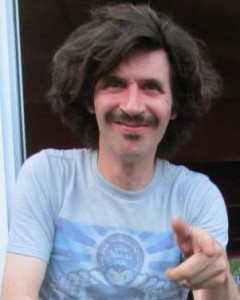
Lavoisier demonstrated with careful measurements that transmutation of water to earth was not possible, but that the sediment observed from boiling water came from the container.
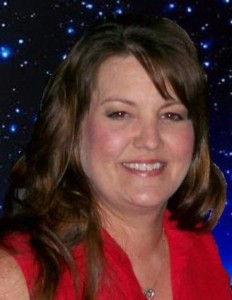
Lavoisier burnt phosphorus and sulfur in air, and proved that the products weighed more than the original materials.
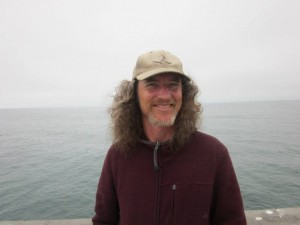
Nevertheless, the weight gained was lost from the air.
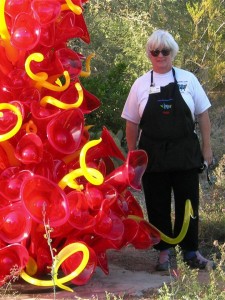
Thus, in 1789, he established the Law of Conservation of Mass, which is also called “Lavoisier’s Law.”
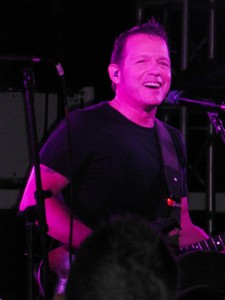
The world’s first ice-calorimeter, was used in the winter of 1782-83, by Antoine Lavoisier and Pierre-Simon Laplace, to determine the heat involved in various chemical changes, calculations which were based on Joseph Black’s prior discovery of latent heat.
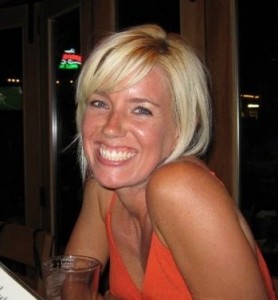
These experiments mark the foundation of thermochemistry.
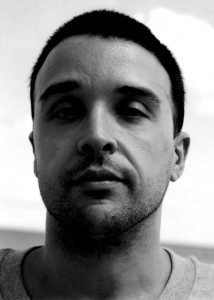
Repeating the experiments of Priestley, he demonstrated that air is composed of two parts, one of which combines with metals to form calxes.
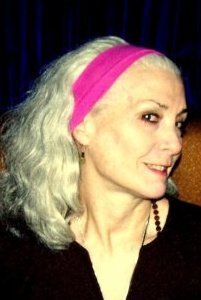
In Considérations Générales sur la Nature des Acides (1778), Lavoisier demonstrated that the “air” responsible for combustion was also the source of acidity.
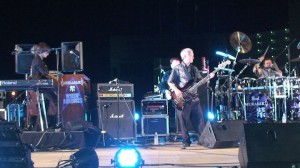
The next year, he named this portion oxygen (Greek for acid-former), and the other azote (Greek for no life).
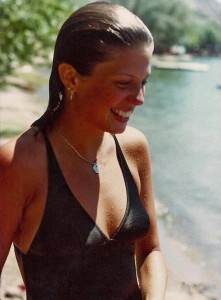
Lavoisier thus has a claim to the discovery of oxygen along with Preistley and Scheele.
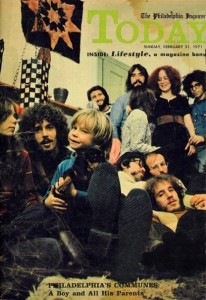
He also discovered that the “inflammable air” discovered by Cavendish, which he termed hydrogen (Greek for water-former), combined with oxygen to produce a dew, as Priestley had reported, which appeared to be water.
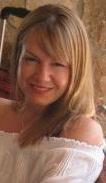
In Reflexions sur le Phlogistique (1783), Lavoisier showed the phlogiston theory of combustion to be inconsistent.
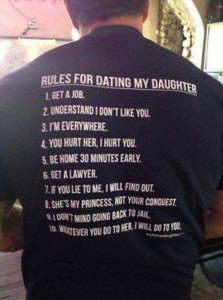
Mikhail Lomonosov independently established a tradition of chemistry in Russia in the 18th century and he also rejected the phlogiston theory, and anticipated the kinetic theory of gases.
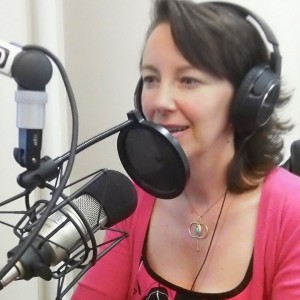
Lomonosov regarded heat as a form of motion, and stated the idea of conservation of matter.
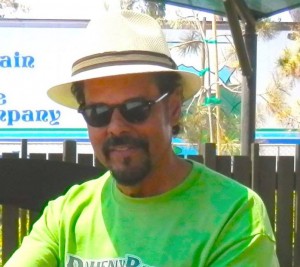
Lavoisier worked with Claude Louis Berthollet and others to devise a system of chemical nomenclature which serves as the basis of the modern system of naming chemical compounds.
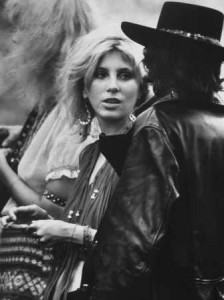
In his Methods of Chemical Nomenclature (1787), Lavoisier invented the system of naming and classification still largely in use today, including names such as sulfuric acid, sulfates and sulfites. Due to these classifications, we are able to ensure that workers who frequently use acidic chemicals are able to work safely. There are many safety protocols in place within companies that use highly dangerous chemicals, you can learn more by reading this Storemasta workplace safety blog.
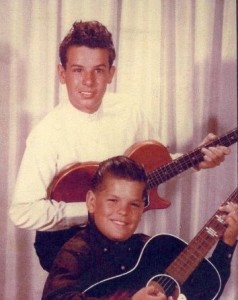
In 1785, Berthollet was the first to introduce the use of chlorine gas as a commercial bleach.
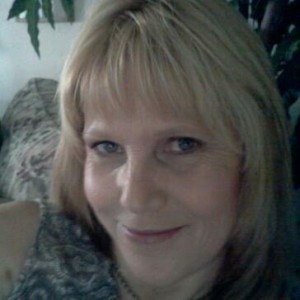
In the same year he first determined the elemental composition of the gas ammonia.
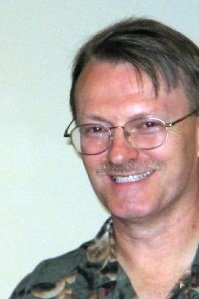
Berthollet first produced a modern bleaching liquid in 1789 by passing chlorine gas through a solution of sodium carbonate.
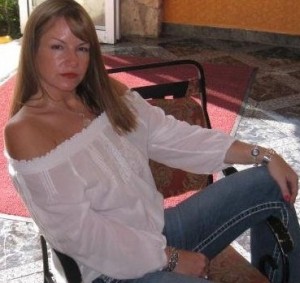
The result was a weak solution of sodium hypochlorite.
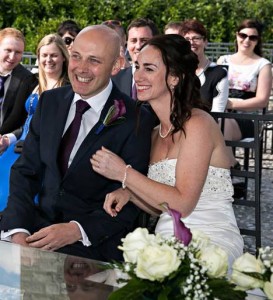
Another strong chlorine oxidant and bleach which he investigated and was the first to produce, potassium chlorate(KClO3), is known as Berthollet’s Salt.
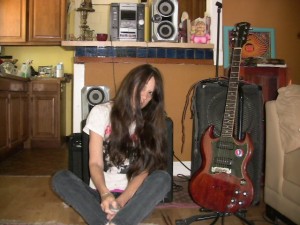
Berthollet is also known for his scientific contributions to theory of chemical equilibria via the mechanism of reverse chemical reactions.
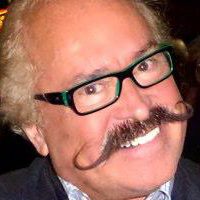
Lavoisier’s Traité Élémentaire de Chimie (Elementary Treatise of Chemistry, 1789) was the first modern chemical textbook, and presented a unified view of new theories of chemistry, contained a clear statement of the Law of Conservation of Mass, and denied the existence of phlogiston.
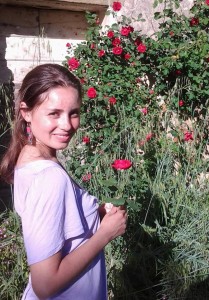
In addition, it contained a list of elements, or substances that could not be broken down further, which included oxygen, nitrogen, hydrogen, phosophorus, mercury, zinc and sulfur.
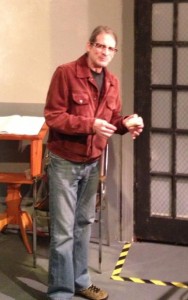
His list, however, also included light and caloric, which he believed to be material substances.
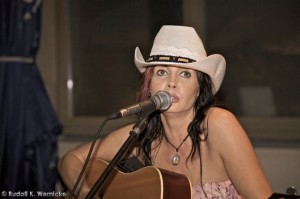
In the work, Lavoisier underscored the observational basis of his chemistry, stating “I have tried…to arrive at the truth by linking up facts; to suppress as much as possible the use of reasoning, which is often an unreliable instrument which deceives us, in order to follow as much as possible the torch of observation and of experiment.”
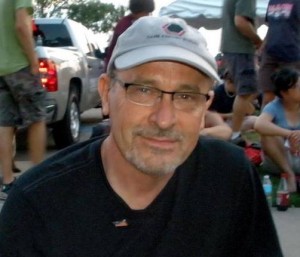
Nevertheless, he believed that the real existence of atoms was philosophically impossible.
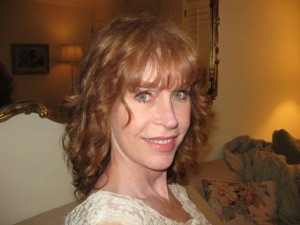
Lavoisier demonstrated that organisms disassemble and reconstitute atmospheric air in the same manner as a burning body.
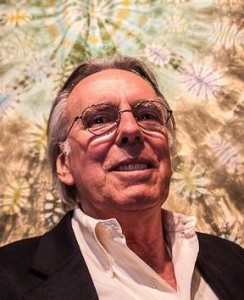
With Pierre-Simon Laplace, Lavoisier used a calorimeter to estimate the heat evolved per unit of carbon dioxide produced.
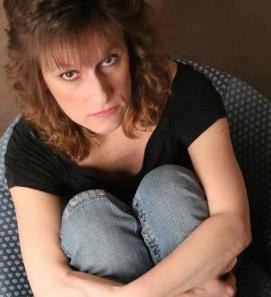
They found the same ratio for a flame and animals, indicating that animals produced energy by a type of combustion.
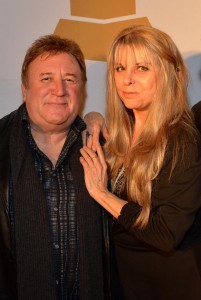
Lavoisier believed in the radical theory, believing that radicals, which function as a single group in a chemical reaction, would combine with oxygen in reactions.
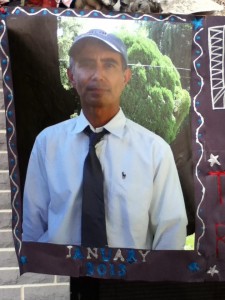
He believed all acids contained oxygen.
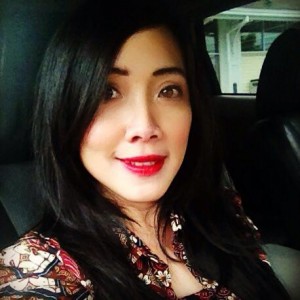
Lavoisier also discovered that a diamond is a crystalline form of carbon.
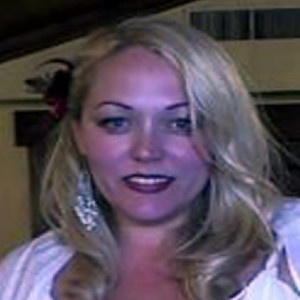
Following Lavoisier’s work, chemistry acquired a strict quantitative nature, allowing reliable predictions to be made.
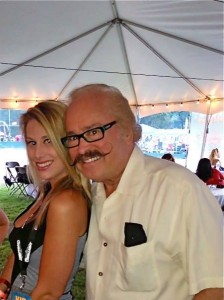
The revolution in chemistry which he brought about was a result of a conscious effort to fit all experiments into the framework of a single theory.
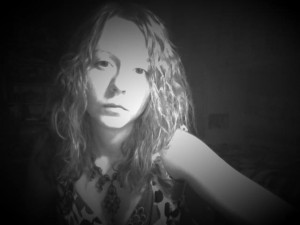
He established the consistent use of chemical balance, used oxygen to overthrow the phlogiston theory, and developed a new system of chemical nomenclature.
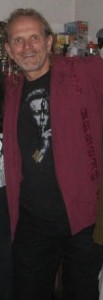
Italian physicist Alessandro Volta constructed a device for accumulating a large charge by a series of inductions and groundings.
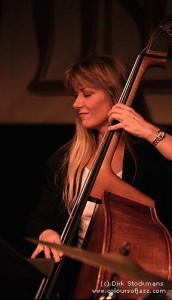
Volta investigated the 1780s discovery “animal electricity” by Luigi Galvani and found that the electric current was generated from the contact of dissimilar metals, and that the frog leg was only acting as a detector.
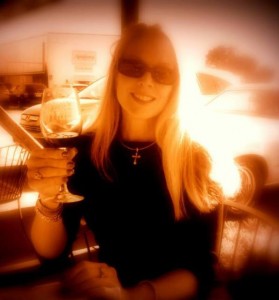
Volta demonstrated in 1794 that when two metals and brine-soaked cloth or cardboard are arranged in a circuit they produce an electric current.
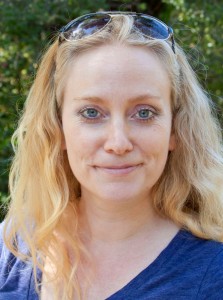
In 1800, Volta stacked several pairs of alternating copper (or silver) and zinc discs (electrodes) separated by cloth or cardboard soaked in vrine (electrolyte) to increase the electrolyte conductivity.
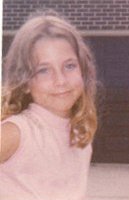
When the top and bottom contacts were connected by a wire, an electric current flowed through the voltaic pile and the connecting wire.
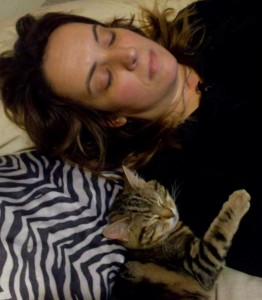
Thus, Volta constructed the first electrical battery to produce electricity.
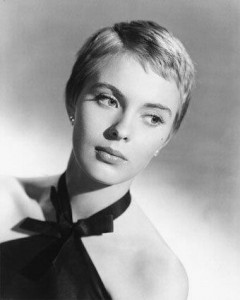
Volta’s method of stacking round plates of copper and zinc separated by disks of cardboard moistened with salt solution was termed a voltaic pile.
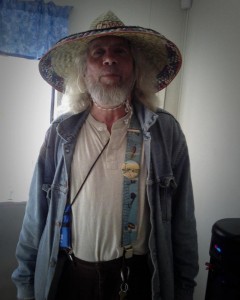
Volta is considered to be the founder of the discipline of electrocheistry.
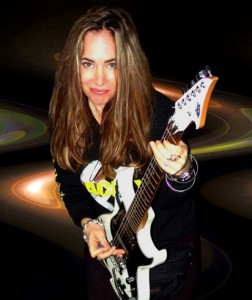
A Galvanic cell (or voltaic cell) is an electrochemical cell that derives electrical energy from spontaneous redox reaction taking place within the cell.
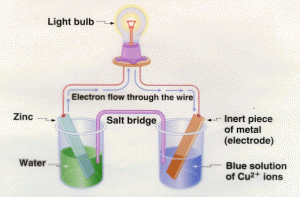
It generally consists of two different metals connected by a salt bridge, or individual half-cells separated by a porous membrane.
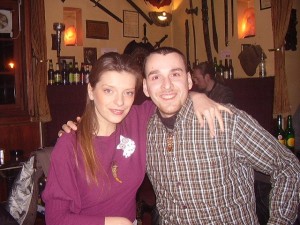
In 1802, French American chemist and industrialist Éleuthère Irénée du Pont, who had learned manufacture of gunpowder and explosives from Antoine Lavoisier, established a gunpowder factory in Delaware known as E.I. du Pont de Nemours and Company.
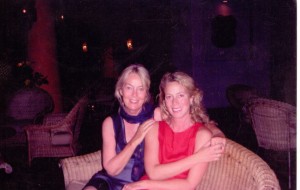
Wanting to make the best powder possible, du Pont was vigilant about the quality of the materials he used.
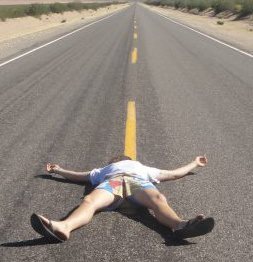
For 32 years, du Pont served as president of E. I. du Pont de Nemours and Company, which eventually grew into one of the largest and most successful companies in America.
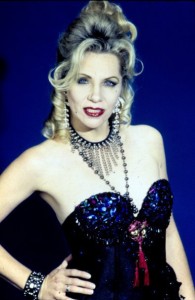
Throughout the 19th century, chemistry was divided between those who followed the atomic theory of John Dalton and those who did not, such as Wilhelm Ostwald and Ernst Mach.
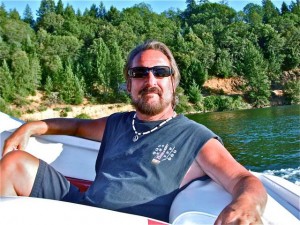
Although such proponents of the atomic theory as Amedeo Avogadro and Ludewig Boltsmann made great advances in explaining the behavior of gases, this dispute was not finally settled until Jean Perrin’s experimental investigation of Einstein’s atomic explanation of Brownian motion in the first decade of the 20th century.
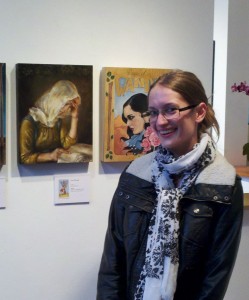
Well before the dispute had been settled, many had already applied the concept of atomism to chemistry.
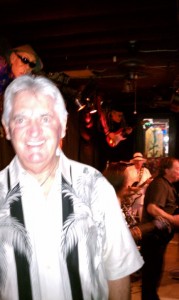
A major example was the ion theory of Svante Arrhenius which anticipated ideas about atomic substructure that did not fully develop until the 20th century.
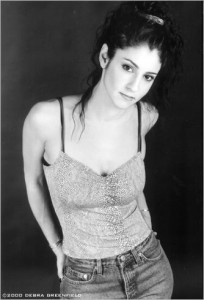
Michael Faraday was another early worker, whose major contribution to chemistry was electrochemistry, in which (among other things) a certain quantity of electricity during electrolysis or electrodeposition of metals was shown to be associated with certain quantities of chemical elements, and fixed quantities of the elements therefore with each other, in specific ratios.
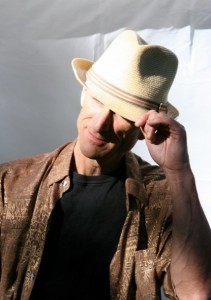
These findings, like those of Dalton’s combining ratios, were early clues to the atomic nature of matter.
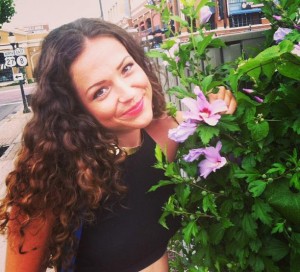
In 1803, English meteorologist and chemistJohn Dalton had proposed Dalton’s law, which describes relationship between the components in a mixture of gases and the relative pressure each contributes to that of the overall mixture.
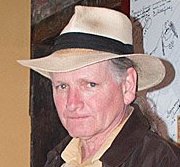
This concept, which John Dalton formulated in 1802, is also known as Dalton’s law of partial pressures.
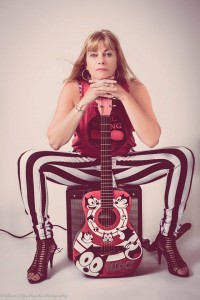
Dalton also proposed an atomic theory in 1803 which stated that all matter was composed of small indivisible particles termed atoms.
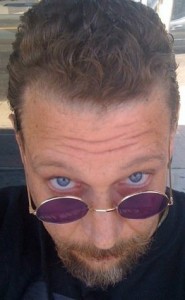
Atoms of a given element possess unique characteristics and weight, and three types of atoms exist: simple (elements), compound (simple molecules), and complex (complex molecules).
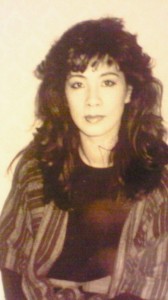
In 1808, Dalton first published New System of Chemical Philosophy (1808-1827), in which he outlined the first modern scientific description of the atomic theory.
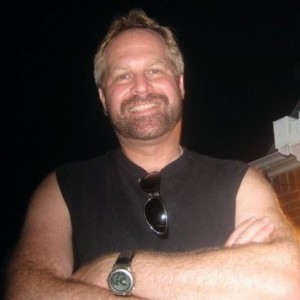
This work identified chemical elements as a specific type of atom, therefore rejecting Newton’s theory of chemical affinities.
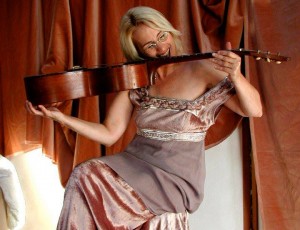
Instead, Dalton inferred proportions of elements in compounds by taking ratios of the weights of reactants, setting the atomic weight of hydrogen to be identically one.
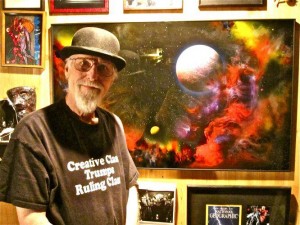
Following Jeremias Benjamin Richer (who was known for introducing the term stoichiometry), John Dalton proposed that chemical elements combine in integral ratios.
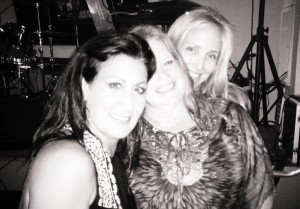
This is known as the law of multiple proportions or Dalton’s law, and Dalton included a clear description of the law in his New System of Chemical Philosophy.
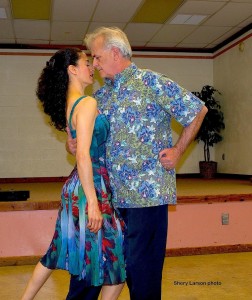
The law of multiple proportions is one of the basic laws of stoichiometry used to establish the atomic theory.
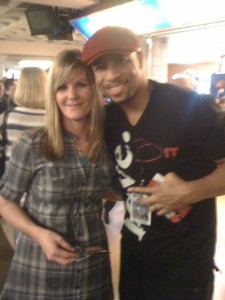
Despite the consideration of atoms as physically real entities and introduction of a system of chemical symbols, New System of Chemical Philosophy devoted almost as much space to the caloric theory as to atomism.
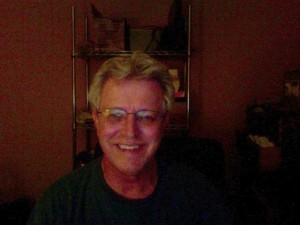
French chemist Joseph Proust proposed the law of definite proportions, which states that elements always combine in small, whole number ratios to form compounds, based on several experiments conducted between 1797 and 1804.
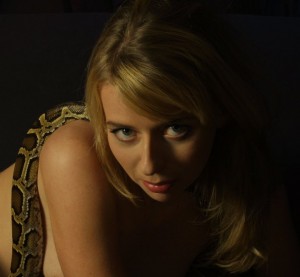
Along with the law of multiple proportions, the law of definite proportions forms the basis of stoichiometry.
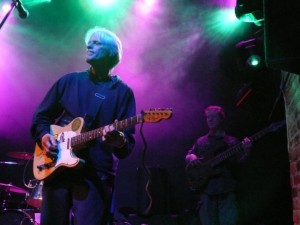
The law of definite proportions and constant composition do not prove that atoms exist, but they are difficult to explain without assuming that chemical compounds are formed when atoms combine in constant proportions.
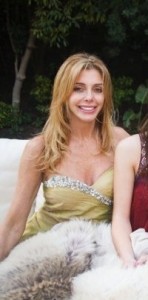
A Swedish chemist and disciple of Dalton, Jöns Jacob Berzelius embarked on a systematic program to try to make accurate and precise quantitative measurements and insure the purity of chemicals.
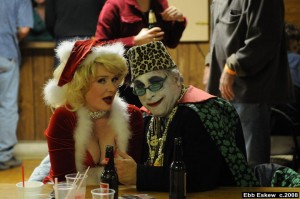
Along with Lavoisier, Boyle, and Dalton, Berzelius is known as one of the fathers of modern chemistry.
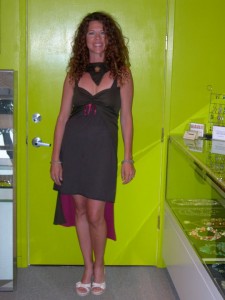
In 1828 he compiled a table of relative atomic weights, where oxygen was assigned the number 100, and which included all of the elements known at the time.
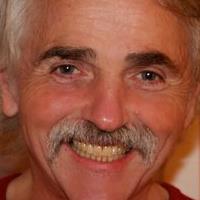
This work provided evidence in favor of Dalton’s atomic theory: that inorganic chemical compounds are composed of atoms combined in whole number amounts.
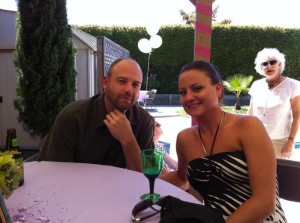
He determined the exact elementary constituents of large numbers of compounds.
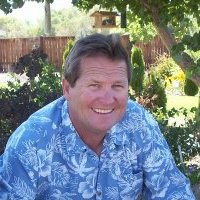
The results strongly confirmed Proust’s Law of Definite Proportions.
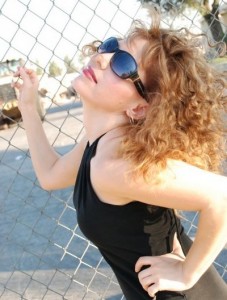
In his weights, he used oxygen as a standard, setting its weight equal to exactly 100. He also measured the weights of 43 elements. In discovering that atomic weights are not integer multiples of the weight of hydrogen, Berzelius also disproved Prout’s hypothesis that elements are built up from atoms of hydrogen.
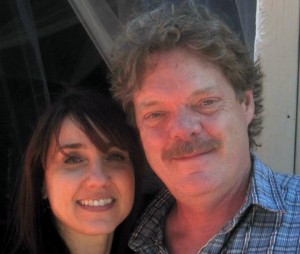
Motivated by his extensive atomic weight determinations and a desire to aid his experiments, Berzelius introduced the classical system of chemical symbols and notation with his 1808 publishing of Lärbok i Kemien, in which elements are abbreviated by one or two letters to make a distinct abbreviation from their Latin name.
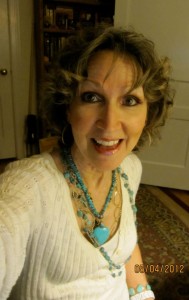
This system of chemical notation-in which the elements were given simple written labels, such as O for oxygen, or Fe for iron, with proportions noted by numbers-is the same basic system used today. The only difference is that instead of the subscript number used today (e.g., H2O), Berzelius used a superscript (H2O).
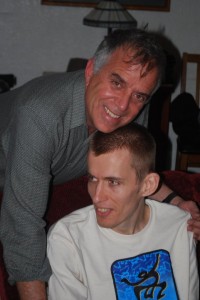
Berzelius is credited with identifying the chemical elements silicon, selenium, thorium and cerium. Students working in Berzelius’s laboratory also discovered lithium and vanadium.
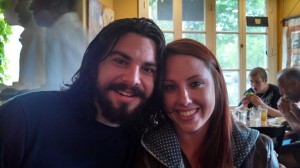
Berzelius developed the radical theory of chemical combination, which holds that reactions occur as stable groups of atoms called radicals are exchanged between molecules.
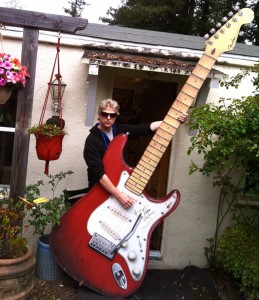
He believed that salts are compounds of an acid and bases, and discovered that the anions in acids would be attracted to a positive electrode (the anode), whereas the cations in a base would be attracted to a negative electrode (the cathode).
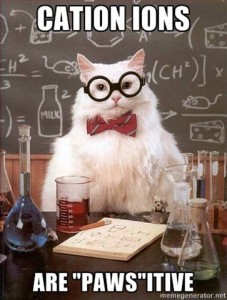
Berzelius did not believe in the Vitalism Theory, but instead in a regulative force which produced organization of tissues in an organism.
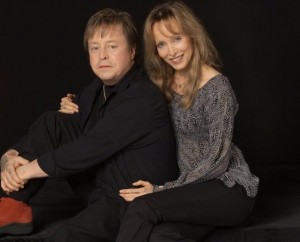
Berzelius is also credited with originating the chemical terms catalysis, polymer, isomer and allotrope, although his original definitions differ dramatically from modern usage. For example, he coined the term “polymer” in 1833 to describe organic compounds which shared identical empirical formulas but which differed in overall molecular weight, the larger of the compounds being described as “polymers” of the smallest. By this long superseded, pre-structural definition, glucose (C6H12O6) was viewed as a polymer of formaldehyde (CH2O).
English chemist Humphry Davy was a pioneer in the field of electrolysis, using Alessandro Volta’s voltaic pile to split up common compounds and thus isolate a series of new elements. He went on to electrolyse molten salts and discovered several new metals, especially sodium and potassium, highly reactive elements known as the alkali metals.
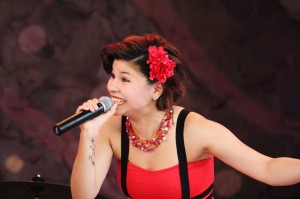
You may remember a clerihew that I quoted about this man: Sir Humphry Davy abominated gravy, and deserved the odium of having discovered sodium.
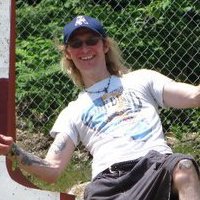
Potassium, the first metal that was isolated by electrolysis, was discovered in 1807 by Davy, who derived it from caustic potash (KOH).
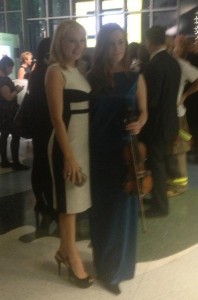
Before the 19th century, no distinction was made between potassium and sodium. Sodium was first isolated by Davy in the same year by passing an electric current through molten sodium hydroxide(NaOH).
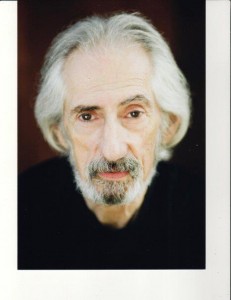
When Davy heard that Berzelius and Pontin prepared calcium amalgam by electrolyzing lime in mercury, he tried it himself. Davy was successful, and discovered calcium in 1808 by electrolyzing a mixture of lime and mercuric oxide. He worked with electrolysis throughout his life and, in 1808, he isolated magnesium, strontium and barium.
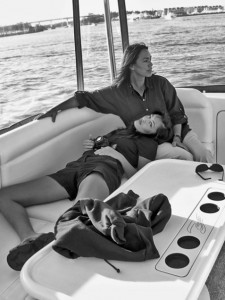
Davy also experimented with gases by inhaling them. This experimental procedure nearly proved fatal on several occasions, but led to the discovery of the unusual effects of nitrous oxide which came to be known as laughing gas. He understood that nitrous oxide had anesthetic properties but didn’t emphasize this fact, and so it was a long time before this compound was used in surgical operations. It is saddening to think of all the needless suffering that happened in the interval between Davy’s discovery of nitrous oxide and its implementation in the medical field.
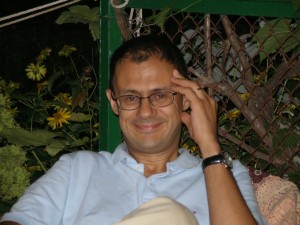
Chlorine was discovered in 1774 by Swedish chemist Carl Wilhelm Scheele, who called it “dephlogisticated marine acid” and mistakenly thought it contained oxygen. Scheele observed several properties of chlorine gas, such as its bleaching effect on litmus, its deadly effect on insects, its yellow-green colour, and the similarity of its smell to that of aqua regia.
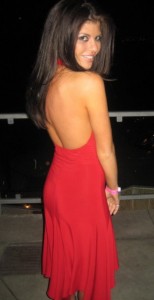
Scheele was unable to publish his findings at the time, and in 1810, chlorine was given its current name by Humphry Davy (derived from the Greek word for green), who insisted that chlorine was in fact an element.
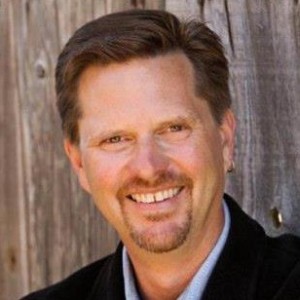
Davy also showed that oxygen could not be obtained from the substance known as oxymuriatic acid (HCl solution). This discovery overturned Lavoisier’s definition of acids as compounds of oxygen.
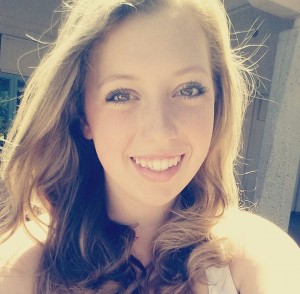
French chemist Joseph Louis Gay-Lussac shared the interest of Lavoisier and others in the quantitative study of the properties of gases.
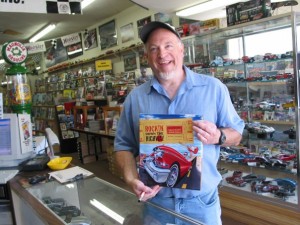
From his first major program of research in 1801–1802, he concluded that equal volumes of all gases expand equally with the same increase in temperature: this conclusion is usually called Charles law (Gay-Lussac gave credit to Jacques Charles, who had arrived at nearly the same conclusion in the 1780s but had not published it).
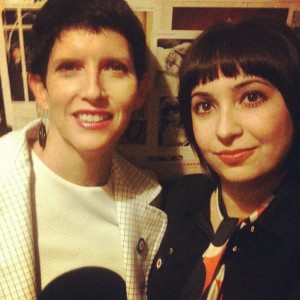
Charles law was independently discovered by John Dalton in 1801, although Dalton’s description was less thorough than Gay-Lussac’s.
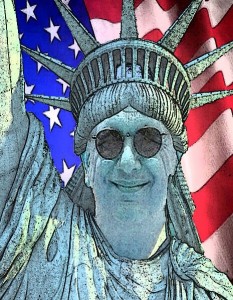
In 1804 Gay-Lussac made several daring ascents of over 7,000 meters above sea level in hydrogen-filled balloons-a feat not equaled for another 50 years-that allowed him to investigate other aspects of gases. Not only did he gather magnetic measurements at various altitudes, but he also took pressure, temperature, and humidity measurements and samples of air, which he later analyzed chemically.
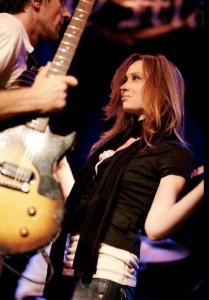
In 1808 Gay-Lussac announced what was probably his single greatest achievement: from his own and others’ experiments he deduced that gases at constant temperature and pressure combine in simple numerical proportions by volume, and the resulting product or products-if gases-also bear a simple proportion by volume to the volumes of the reactants. In other words, gases under equal conditions of temperature and pressure react with one another in volume ratios of small whole numbers. This conclusion subsequently became known as Gay-Lussac’s law or the Law of Combining Volumes.
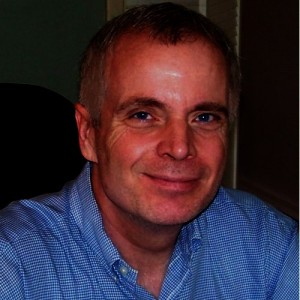
With his fellow professor at the École Polytechnique, Louis Jacques Thénard, Gay-Lussac also participated in early electrochemical research, investigating the elements discovered by its means. Among other achievements, they decomposed boric acid by using fused potassium, thus discovering the element boron.
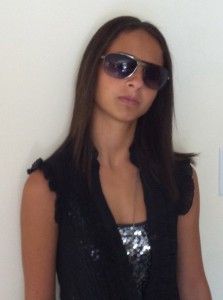
The two also took part in contemporary debates that modified Lavoisier’s definition of acids and furthered his program of analyzing organic compounds for their oxygen and hydrogen content.
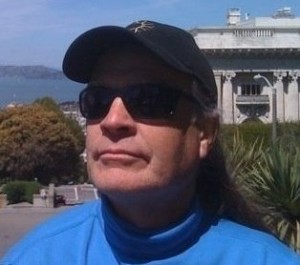
The element iodine was discovered by French chemist Bernard Courtois in 1811. Courtois gave samples to his friends,Charles Bernard Desormes (1777-1862) and Nicolas Clément (1779–1841), to continue research. He also gave some of the substance to Gay-Lussac and to physicist André-Marie Ampère.
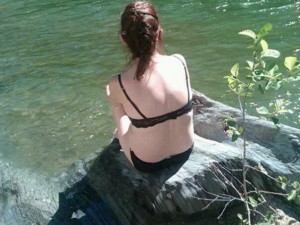
On December 6, 1813, Gay-Lussac announced that the new substance was either an element or a compound of oxygen. It was Gay-Lussac who suggested the name “iode”, from the Greek word ????? (iodes) for violet (because of the color of iodine vapor).
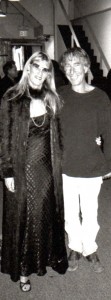
Ampère had given some of his sample to Humphry Davy. Davy did some experiments on the substance and noted its similarity to chlorine. Davy sent a letter dated December 10 to the Royal Society of London stating that he had identified a new element. Arguments erupted between Davy and Gay-Lussac over who identified iodine first, but both scientists acknowledged Courtois as the first to isolate the element.
In 1815, Humphry Davy invented the Davy lamp, which allowed coal miners to work safely in the presence of flammable gases. There had been many mining explosions caused by firedamp or methane, often ignited by open flames of the lamps then used by miners. Davy thought of using an iron gauze to enclose a lamp’s flame, and so prevent the methane burning inside the lamp from passing out to the general atmosphere.
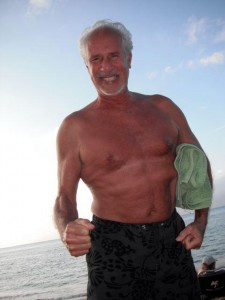
Although the idea of the safety lamp had already been demonstrated by William Reid Clanny and by the then unknown (but later very famous) engineer George Stephenson, Davy’s use of wire gauze to prevent the spread of flame was used by many other inventors in their later designs.
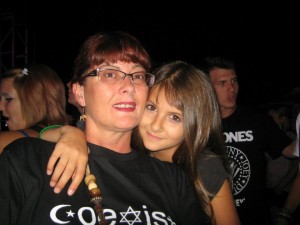
There was some discussion as to whether Davy would have discovered the principles behind his lamp without the help of the work of Smithson Tennant, but it was generally agreed that the work of both men had been independent. Davy refused to patent the lamp, and its invention led to his being awarded the Rumford medal in 1816.
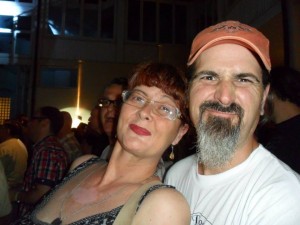
After Dalton published his atomic theory in 1808, certain of his central ideas were soon adopted by most chemists. However, uncertainty persisted for half a century about how atomic theory was to be configured and applied to concrete situations. Chemists in different countries developed several different incompatible atomistic systems.
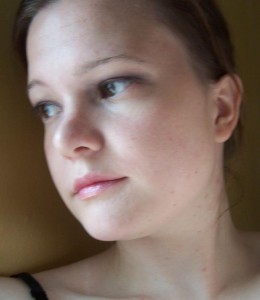
A paper that suggested a way out of this difficult situation was published as early as 1811 by the Italian physicist Amedeo Avogadro (1776-1856), who hypothesized that equal volumes of gases at the same temperature and pressure contain equal numbers of molecules, from which it followed that relative molecular weights of any two gases are the same as the ratio of the densities of the two gases under the same conditions of temperature and pressure.
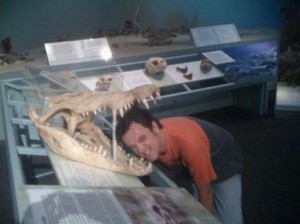
Avogadro also reasoned that simple gases were not formed of solitary atoms but were instead compound molecules of two or more atoms. Thus Avogadro was able to overcome the difficulty that Dalton and others had encountered when Gay-Lussac reported that above 100 °C the volume of water vapor was twice the volume of the oxygen used to form it. According to Avogadro, the molecule of oxygen had split into two atoms in the course of forming water vapor.
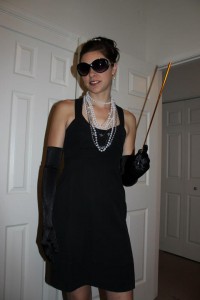
Avogadro’s hypothesis was neglected for half a century after it was first published. Many reasons for this neglect have been cited, including some theoretical problems, such as Jöns Jacob Berzelius’s “dualism,” which asserted that compounds are held together by the attraction of positive and negative electrical charges, making it inconceivable that a molecule composed of two electrically similar atoms-as in oxygen-could exist.
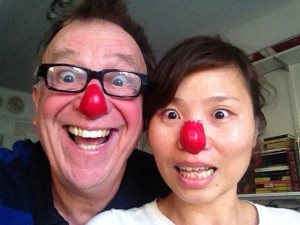
An additional barrier to acceptance of Avogadro’s hypothesis was the fact that many chemists were reluctant to adopt physical methods (such as vapour-density determinations) to solve their problems. By mid-century, however, some leading figures had begun to view the chaotic multiplicity of competing systems of atomic weights and molecular formulas as intolerable. Moreover, purely chemical evidence began to mount that suggested Avogadro’s approach might be right after all.
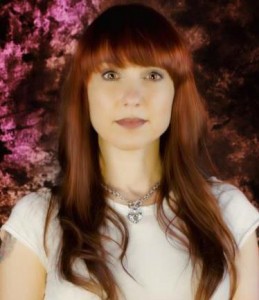
During the 1850s, younger chemists, such as Alexander Williamson in England, Charles Gerhardt and Charles-Adolphe Wurtz in France, and August Kekulé in Germany, began to advocate reforming theoretical chemistry to make it consistent with Avogadrian theory.
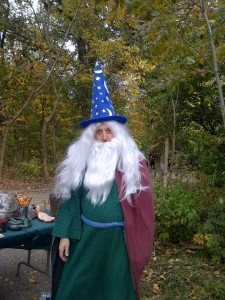
In 1825, Friedrich Wöhler and Justus von Liebig performed the first confirmed discovery and explanation of isomers earlier named by Berzelius.
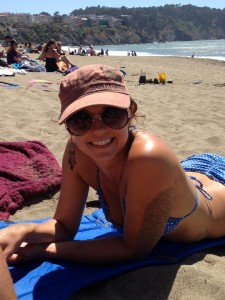
Working with cyanic acid and fulminic acid, they correctly deduced that isomerism was caused by differing arrangements of atoms within a molecular structure.
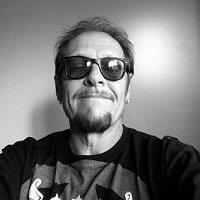
In 1827, William Prout classified biomolecules into their modern groupings: carbohydrates, proteins and lipids.
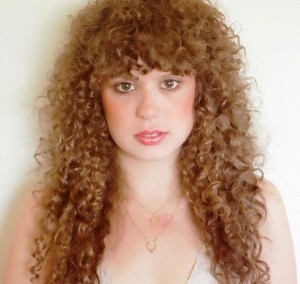
After the nature of combustion was settled, another dispute, this one concerning vitalism and the essential distinction between organic and inorganic substances, began. The vitalism question was revolutionized in 1828 when Friedrich Wöhler synthesized urea, thereby establishing that organic compounds could be produced from inorganic starting materials and disproving the theory of vitalism. Never before had an organic compound been synthesized from inorganic material.
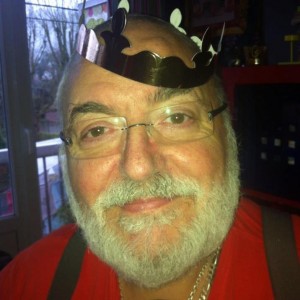
This opened a new research field in chemistry, and by the end of the 19th century, scientists were able to synthesize hundreds of organic compounds, the most important among them being mauve, magenta and other synthetic dyes, as well as the widely used drug aspirin. You have probably heard it said of aspirin, that, were it invented today, you would need a prescription for it, since its uses are manifold.
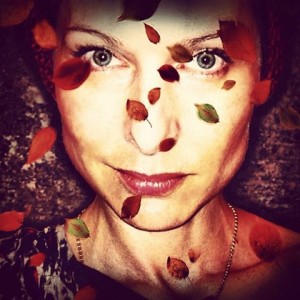
The discovery of the artificial synthesis of urea contributed greatly to the theory of isomerism, as the empirical chemical formulas for urea and ammonium cyanate are identical.
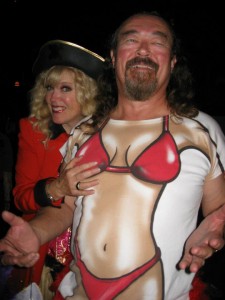
In 1832, Friedrich Wöhler and Justus von Liebig discovered and explained functional groups and radicals in relation to organic chemistry, as well as first synthesizing benzaldehyde.
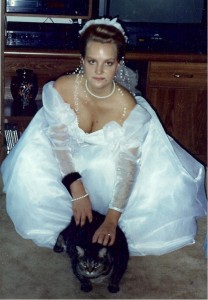
Liebig, a German chemist, made major contributions to agricultural and biological chemistry, and worked on the organization of organic chemistry, and he is considered the “father of the fertilizer industry” for his discovery of nitrogen as an essential plant nutrient, and his formulation of the Law of the Minimum which described the effect of individual nutrients on crops.
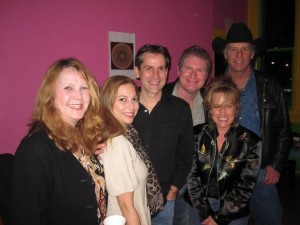
In 1840, Germain Hess proposed Hess’ law, an early statement of the law of conservation of energy, which establishes that energy changes in a chemical process depend only on the states of the starting and product materials and not on the specific pathway taken between the two states.
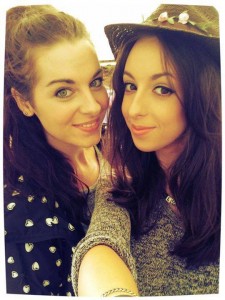
In 1847, Hermann Kolbe obtained acetic acid from completely inorganic sources, further disproving vitalism.
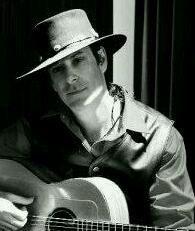
In 1848, William Thomson, 1st Baron Kelvin (commonly known as Lord Kelvin), established the concept of absolute zero, the temperature at which all molecular motion ceases.
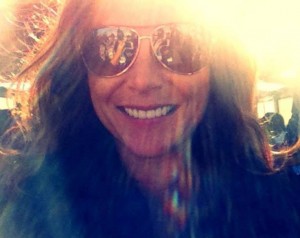
In 1849, Louis Pasteur discovered that the racemic form of tartaric acid is a mixture of the levorotatory and dextrotatory forms, thus clarifying the nature of optical rotation and advancing the field of stereochemistry.
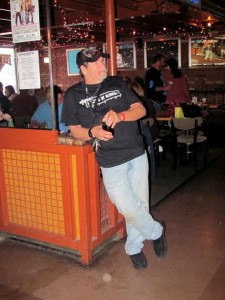
In 1852, August Beer proposed Beer’s law, which explains the relationship between the composition of a mixture and the amount of light it will absorb. Based partly on earlier work by Pierre Bouguer and Johann Heinrich Lambert, Beer’s law established the analytical technique known as spectrophotometry.
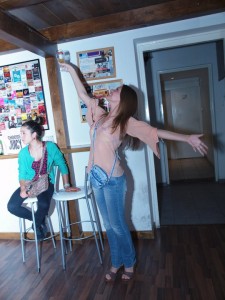
In 1855, Benjaman Silliman, Jr. pioneered methods of petroleum cracking which made the entire modern petrochemical industry possible, so we love him, right?
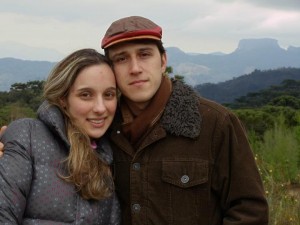
Avogadro’s hypothesis was that that equal volumes of gases at the same temperature and pressure contain equal numbers of molecules, from which it followed that relative molecular weights of any two gases are the same as the ratio of the densities of the two gases under the same conditions of temperature and pressure.
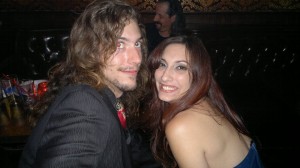
This hypothesis began to gain broad appeal among chemists only after his compatriot and fellow scientist Stanislao Cannizzarro demonstrated its value in 1858, two years after Avogadro’s death.
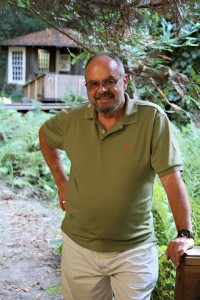
Cannizzaro’s chemical interests had originally centered on natural products and on reactions of aromatic compounds
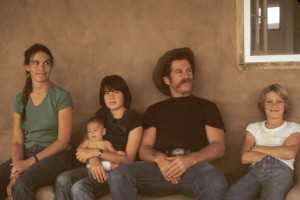
In 1853 he discovered that when benzaldehyde is treated with concentrated base, both benzoic acid and benzyl alcohol are produced, a phenomenon known today as the Cannizzaro reaction. In his 1858 pamphlet, Cannizzaro showed that a complete return to the ideas of Avogadro could be used to construct a consistent and robust theoretical structure that fit nearly all of the available empirical evidence. For instance, he pointed to evidence that suggested that not all elementary gases consist of two atoms per molecule-some were monoatomic, but most were diatomic, and a few were even more complex.
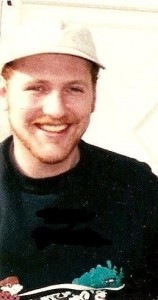
Another point of contention had been the formulas for compounds of the alkali metals(such as sodium) and the alkaline earth metals (such as calcium), which, in view of their striking chemical analogies, most chemists had wanted to assign to the same formula type.
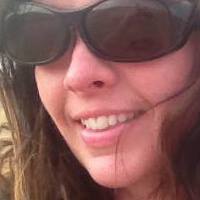
Cannizzaro argued that placing these metals in different categories had the beneficial result of eliminating certain anomalies when using their physical properties to deduce atomic weights. Unfortunately, Cannizzaro’s pamphlet was published initially only in Italian and had little immediate impact.
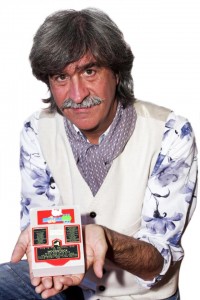
The real breakthrough came with an international chemical congress held in the German town of Karlsruhe in September 1860, at which most of the leading European chemists were present. The Karlsruhe Congress had been arranged by Kékule, Wurtz, and a few others who shared Cannizzaro’s sense of the direction chemistry should go.
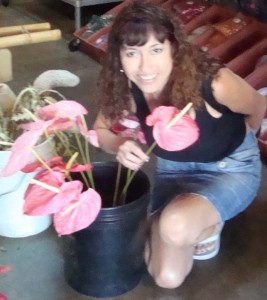
Speaking in French (as everyone there did), Cannizzaro made an indelible impression on the assembled body. Moreover, his friend Angelo Pavesi distributed Cannizzaro’s pamphlet to attendees at the end of the meeting; more than one chemist later wrote of the decisive impression the reading of this document provided.
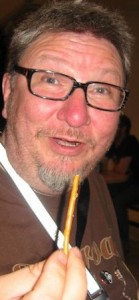
For instance, Lothar Meyerlater wrote that on reading Cannizzaro’s paper, “The scales seemed to fall from my eyes.” Cannizzaro thus played a crucial role in winning the battle for reform. The system advocated by him, and soon thereafter adopted by most leading chemists, is substantially identical to what is still used today.
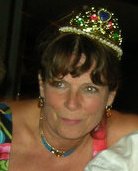
In 1856, Sir William Henry Perkin, age 18, given a challenge by his professor, August Wilhelm von Hofmann, sought to synthesize quinine, the anti-malaria drug from coal tar. In one attempt, Perkin oxidized aniline using potassium dichromate, whose toluidine impurities reacted with the aniline and yielded a black solid-suggesting a “failed” organic synthesis.
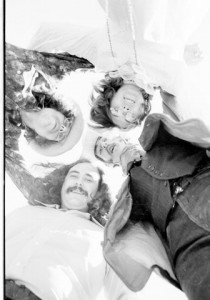
As he was cleaning the flask with alcohol, Perkin noticed purple portions of the solution: a byproduct of the attempt was the first synthetic dye, known as mauveine or Perkin’s mauve. Perkin’s discovery is the foundation of the dye synthesis industry, one of the earliest successful chemical industries.
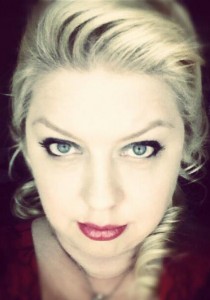
German chemist August Kekulé von Stradonitz’s most important single contribution was his structural theory of organic composition, outlined in two articles published in 1857 and 1858 and treated in great detail in the pages of his extraordinarily popular Lehrbuch der organischen Chemie (“Textbook of Organic Chemistry”), the first installment of which appeared in 1859 and gradually extended to four volumes.
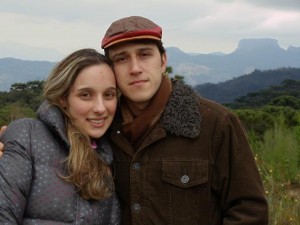
Kekulé argued that tetravalent carbon atoms, that is, carbon forming exactly four chemical bonds, could link together to form what he called a “carbon chain” or a “carbon skeleton,” to which other atoms with other valences (such as hydrogen, oxygen, nitrogen, and chlorine) could join. He was convinced that it was possible for the chemist to specify this detailed molecular architecture for at least the simpler organic compounds known in his day.
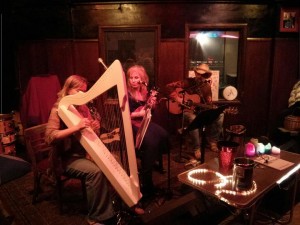
Kekulé was not the only chemist to make such claims in this era. The Scottish chemist Archibald Scott Couper published a substantially similar theory nearly simultaneously, and the Russian chemist Aleksandr Butlerov did much to clarify and expand structure theory. However, it was predominantly Kekule’s ideas that prevailed in the chemical community.
British chemist and physicist William Crookes is noted for his cathode ray studies, fundamental in the development of atomic physics.
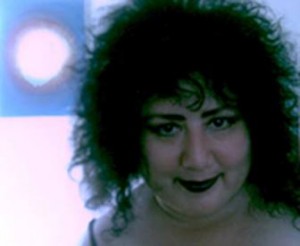
His researches on electrical discharges through a rarefied gas led him to observe the dark space around the cathode, now called the Crookes dark space. He demonstrated that cathode rays travel in straight lines and produce phosphorescence and heat when they strike certain materials.
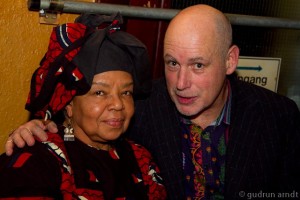
A pioneer of vacuum tubes, Crookes invented the Crookes tube – an early experimental discharge tube, with partial vacuum with which he studied the behavior of cathode rays.
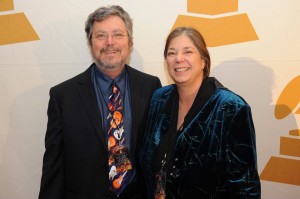
With the introduction of spectrum analysis by Robert Bunsen and Gustav Kirchhoff (1859-1860), Crookes applied the new technique to the study of selenium compounds. Bunsen and Kirchoff had previously used spectroscopy as a means of chemical analysis to discover caesium and rubidium.
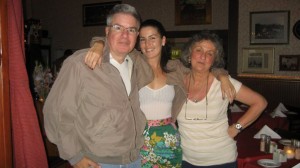
In 1861, Crookes used this process to discover thallium in some seleniferous deposits. He continued work on that new element, isolated it, studied its properties, and in 1873 determined its atomic weight. During his studies of thallium, Crookes discovered the principle of the Crookes radiometer a device that converts light radiation into rotary motion. The principle of this radiometer has found numerous applications in the development of sensitive measuring instruments.
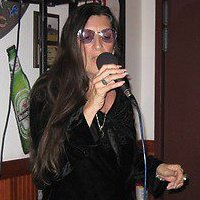
In 1862,Alexander Parkes exhibited Parkesine, one of the earliest synthetic polymers, at the International Exhibition in London. This discovery formed the foundation of the modern plastics industry.
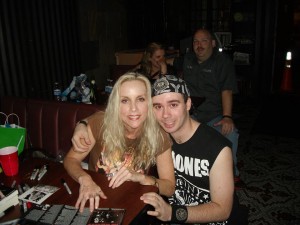
In 1864, Cato Maximilian Guldberg and Peter Waage, building on Claude Louis Berthollet’s ideas, proposed the law of mass action.
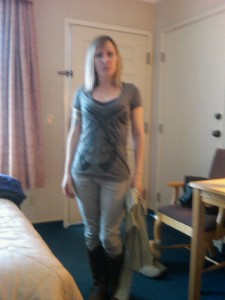
In 1865, Johann Josef Loschmidt determined the exact number of molecules in a mole, later named Avogadro’s number.
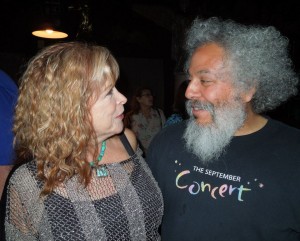
In 1865, August Kekulé, based partially on the work of Loschmidt and others, established the structure of benzene as a six carbon ring with alternating single and double bonds. Kekulé’s novel proposal for benzene’s cyclic structure was much contested but was never replaced by a superior theory. This theory provided the scientific basis for the dramatic expansion of the German chemical industry in the last third of the 19th century.
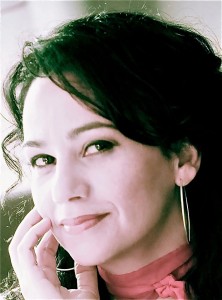
Today, the large majority of known organic compounds are aromatic, and all of them contain at least one hexagonal benzene ring of the sort that Kekulé advocated. Kekulé is also famous for having clarified the nature of aromatic compounds, which are compounds based on the benzene molecule.
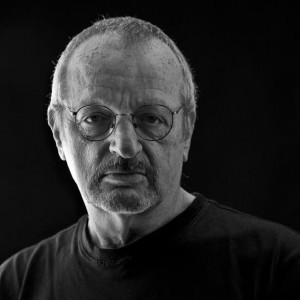
In 1865, Adolf von Baeyer began work on indigo dye, a milestone in modern industrial organic chemistry which revolutionized the dye industry.
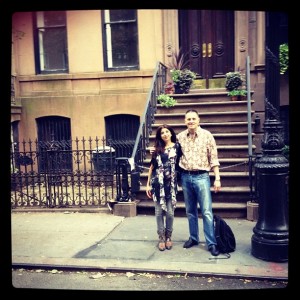
Swedish chemist and inventor Alfred Nobel found that when nitroglycerin was incorporated in an absorbent inert substance like kieselguhr (diatomaceous earth) it became safer and more convenient to handle, and this mixture he patented in 1867 as dynamite. Nobel later on combined nitroglycerin with various nitrocellulose compounds, similar to collodion, but settled on a more efficient recipe combining another nitrate explosive, and obtained a transparent, jelly-like substance, which was a more powerful explosive than dynamite.
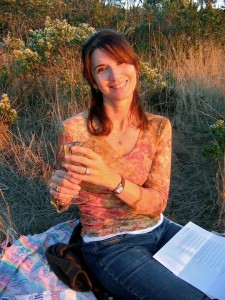
Gelignite, or blasting gelatin, as it was named, was patented in 1876; and was followed by a host of similar combinations, modified by the addition of potassium nitrate and various other substances.
An important breakthrough in making sense of the list of known chemical elements (as well as in understanding the internal structure of atoms) was Dmitri Mendeleev’s development of the first modern periodic table, or the periodic classification of the elements.
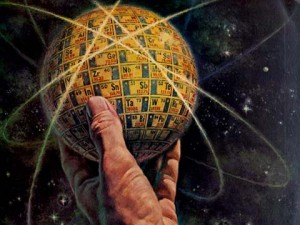
Mendeleev, a Russian chemist, felt that there was some type of order to the elements and he spent more than thirteen years of his life collecting data and assembling the concept, initially with the idea of resolving some of the disorder in the field for his students. Mendeleev found that, when all the known chemical elements were arranged in order of increasing atomic weight, the resulting table displayed a recurring pattern, or periodicity, of properties within groups of elements.
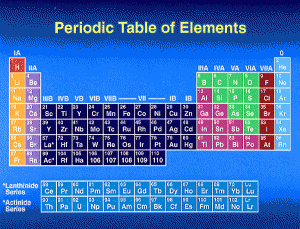
Mendeleev’s law allowed him to build up a systematic periodic table of all the 66 elements then known based on atomic mass, which he published in Principles of Chemistry in 1869. His first Periodic Table was compiled on the basis of arranging the elements in ascending order of atomic weight and grouping them by similarity of properties.
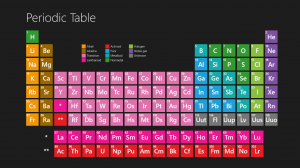
Mendeleev had such faith in the validity of the periodic law that he proposed changes to the generally accepted values for the atomic weight of a few elements and, in his version of the periodic table of 1871, predicted the locations within the table of unknown elements together with their properties.
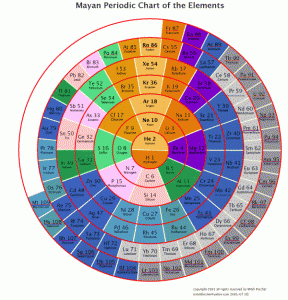
Mendeleev even predicted the likely properties of three yet-to-be-discovered elements, which he called ekaboron (Eb), ekaaluminium (Ea), and ekasilicon (Es), which proved to be good predictors of the properties of scandium, gallium and germanium, respectively, which each fill the spot in the periodic table assigned by Mendeleev.
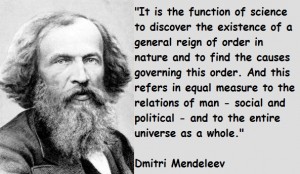
At first the periodic system did not raise interest among chemists. However, with the discovery of the predicted elements, notably gallium in 1875, scandium in 1879, and germanium in 1886, it began to win wide acceptance. The subsequent proof of many of his predictions within his lifetime brought fame to Mendeleev as the founder of the periodic law.
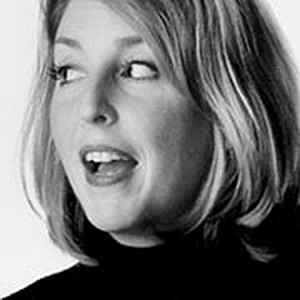
This organizational system of Mendeleev’s surpassed earlier attempts at classification by Alexandre-Émile Béguyer de Chancourtois, who published the telluric helix, an early, three-dimensional version of the periodic table of the elements in 1862, by John Newlands, who proposed the law of octaves (a precursor to the periodic law) in 1864, and by Lothar Meyer, who developed an early version of the periodic table with 28 elements organized by valencein 1864.
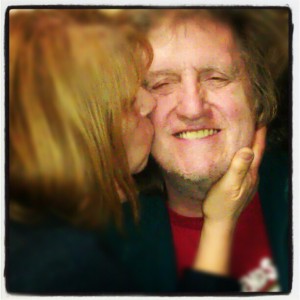
Mendeleev’s table did not include any of the noble gases, however, which had not yet been discovered. Gradually the periodic law and table became the framework for a great part of chemical theory. By the time Mendeleyev died in 1907, he enjoyed international recognition and had received distinctions and awards from many countries.
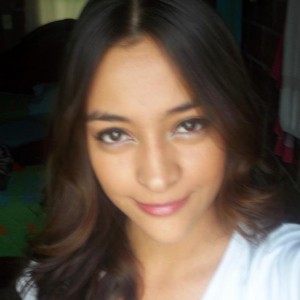
In 1873, Jacobus Henricus van’t Hoff and Joseph Achille Le Bel, working independently, developed a model of chemical bonding that explained the chirality experiments of Pasteur and provided a physical cause for optical activity in chiral compounds.
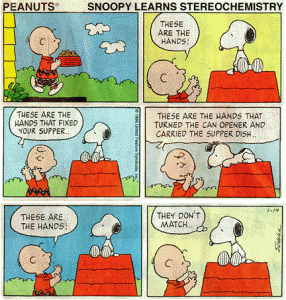
Van ‘t Hoff’s publication, called Voorstel tot Uitbreiding der Tegenwoordige in de Scheikunde gebruikte Structuurformules in de Ruimte (Proposal for the development of 3-dimensional chemical structural formulae) and consisting of twelve pages text and one page diagrams, gave the impetus to the development of stereochemistry.
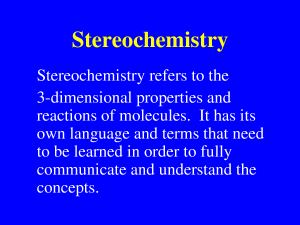
The concept of the “asymmetrical carbon atom”, dealt with in this publication, supplied an explanation of the occurrence of numerous isomers, inexplicable by means of the then current structural formulae. At the same time he pointed out the existence of relationship between optical activity and the presence of an asymmetrical carbon atom.
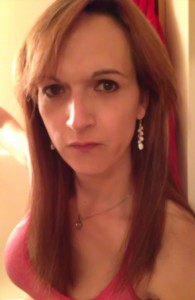
American mathematical physicist J. Willard Gibb’s work on the applications of thermodynamics was instrumental in transforming physical chemistry into a rigorous deductive science. During the years from 1876 to 1878, Gibbs worked on the principles of thermodynamics, applying them to the complex processes involved in chemical reactions.
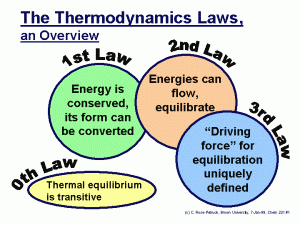
Gibbs discovered the concept of chemical potential, or the “fuel” that makes chemical reactions work. In 1876 he published his most famous contribution, On the Equilibrium of Heterogeneous Substances, a compilation of his work on thermodynamics and physical chemistry which laid out the concept of free energy to explain the physical basis of chemical equilibria.
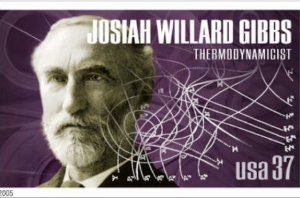
In these essays were the beginnings of Gibbs’ theories of phases of matter: he considered each state of matter a phase, and each substance a component. Gibbs took all of the variables involved in a chemical reaction – temperature, pressure, energy, volume, and entropy – and included them in one simple equation known as Gibbs’ phase rule.
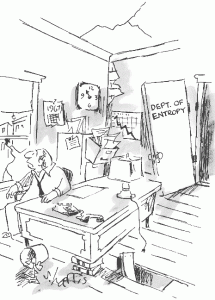
Within this paper was perhaps his most outstanding contribution, the introduction of the concept free energy, now universally called Gibbs’ free energy in his honor. The Gibbs free energy relates the tendency of a physical or chemical system to simultaneously lower its energy and increase its disorder, or entropy, in a spontaneous natural process.
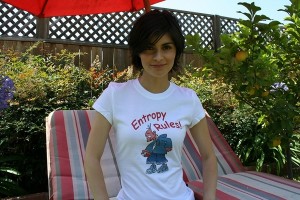
Gibbs’s approach allows a researcher to calculate the change in free energy in the process, such as in a chemical reaction, and how fast it will happen. Since virtually all chemical processes and many physical ones involve such changes, his work has significantly impacted both the theoretical and experiential aspects of these sciences.
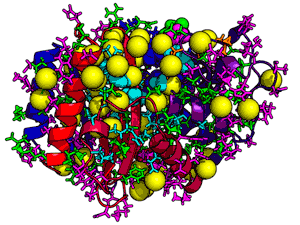
In 1877, Ludwig Boltzmann established statistical derivations of many important physical and chemical concepts, including entropy, and distributions of molecular velocities in the gas phase. Together with Boltzmann and James Clerk Maxwell, Gibbs created a new branch of theoretical physics called statistical mechanics (a term that he coined), explaining the laws of thermodynamics as consequences of the statistical properties of large ensembles of particles.
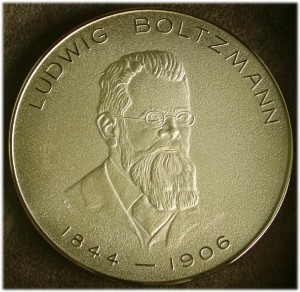
Gibbs also worked on the application of Maxwell’s equations to problems in physical optics. Gibbs’s derivation of the phenomenological laws of thermodynamics from the statistical properties of systems with many particles was presented in his highly-influential textbook Elementary Principles in Statistical Mechanics, published in 1902, a year before his death.
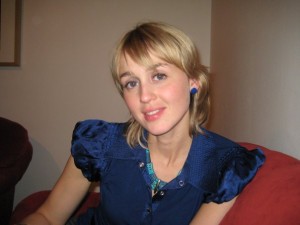
In that work, Gibbs reviewed the relationship between the laws of thermodynamics and statistical theory of molecular motions. The overshooting of the original function by partial sums of Fourier series at points of discontinuity is known as the Gibbs phenomenon.
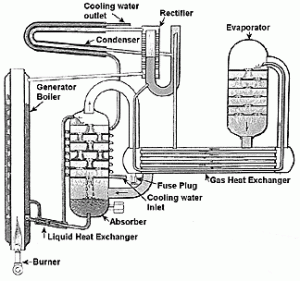
German engineer Carl von Linde’s invention of a continuous process of liquefying gases in large quantities formed a basis for the modern technology of refrigerationand provided both impetus and means for conducting scientific research at low temperatures and very high vacuums.
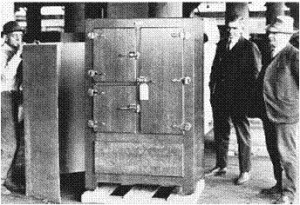
Von Linde developed a methyl ether refrigerator (1874) and an ammonia refrigerator (1876). Though other refrigeration units had been developed earlier, Linde’s were the first to be designed with the aim of precise calculations of efficiency.
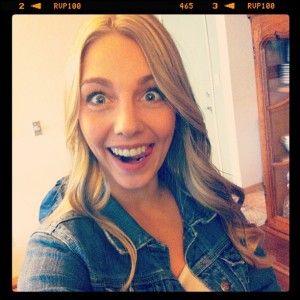
In 1895 he set up a large-scale plant for the production of liquid air, and six years later he developed a method for separating pure liquid oxygen from liquid air that resulted in widespread industrial conversion to processes utilizing oxygen (e.g., in steel manufacture).
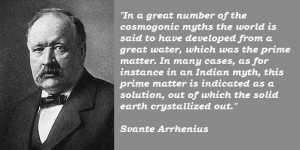
In 1883, Svante Arrhenius developed an ion theory to explain conductivity in electrolytes.
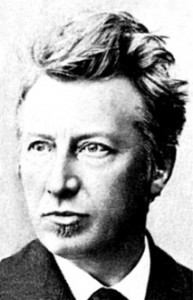
In 1884, Jacobus Henricus van’t Hoff published Études de Dynamique chimique (Studies in Dynamic Chemisty), a seminal study on chemical kinetics. In this work, van ‘t Hoff entered for the first time the field of physical chemistry. Of great importance was his development of the general thermodynamic relationship between the heat of conversion and the displacement of the equilibrium as a result of temperature variation.
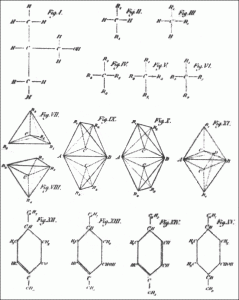
At constant volume, the equilibrium in a system will tend to shift in such a direction as to oppose the temperature change which is imposed upon the system.
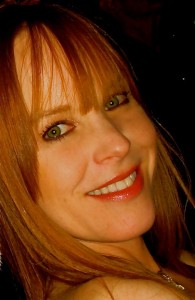
Thus, lowering the temperature results in heat development while increasing the temperature results in heat absorption. This principle of mobile equilibrium was subsequently (1885) put in a general form by Henry Louis Le Chatelier, who extended the principle to include compensation, by change of volume, for imposed pressure changes.
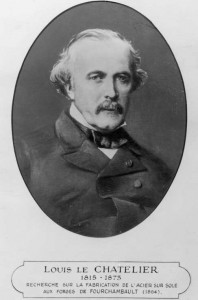
The van ‘t Hoff-Le Chatelier principle, or simply Le Chatelier’s principle explains the response of dynamic chemical equilibria to external stresses.
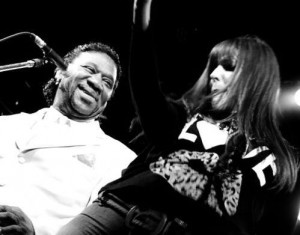
In 1884,Hermann Emil Fischer proposed the structure of purine, a key structure in many biomolecules, which he later synthesized in 1898. He also began work on the chemistry of glucose and related sugars.
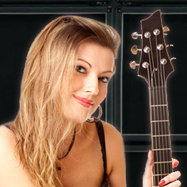
In 1885 Eugene Goldstein named the cathode ray, later discovered to be composed of electrons, and the canal ray later discovered to be positive hydrogen ions that had been stripped of their electrons in a cathode ray tube. These would later be named protons.
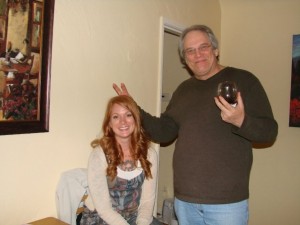
The year 1885 also saw the publishing of J. H. van ‘t Hoff’s L’Équilibre chimique dans les Systèmes gazeux ou dissous à I’État dilué (Chemical equilibria in gaseous systems or strongly diluted solutions), which dealt with this theory of dilute solutions. Here he demonstrated that the osmotic pressure in solutions which are sufficiently dilute is proportionate to the concentration and the absolute temperature so that this pressure can be represented by a formula which only deviates from the formula for gas pressure by a coefficient i.
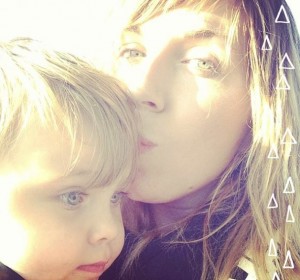
Van’t Hoff also determined the value of i by various methods, for example by means of the vapor pressure and François-Marie Raoult’s results on the lowering of the freezing point. Thus van ‘t Hoff was able to prove that thermodynamic laws are not only valid for gases, but also for dilute solutions.
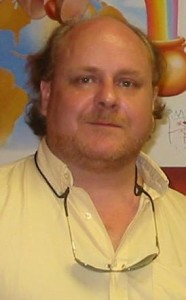
His pressure laws, given general validity by the electrolytic dissociation theory of Arrhenius (1884-1887), the first foreigner who came to work with him in Amsterdam (1888), are considered the most comprehensive and important in the realm of natural sciences.
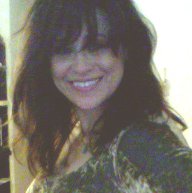
In 1893, Alfred Werner discovered the octahedral structure of cobalt complexes, thus establishing the field of coordination chemistry.
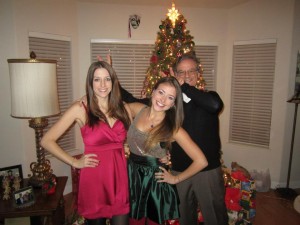
The most celebrated discoveries of Scottish chemist William Ramsay were made in inorganic chemistry. Ramsay was intrigued by the British physicist John Strutt, 3rd Baron Rayleigh’s 1892 discovery that the atomic weight of nitrogen found in chemical compounds was lower than that of nitrogen found in the atmosphere. He ascribed this discrepancy to a light gas included in chemical compounds of nitrogen, while Ramsay suspected a hitherto undiscovered heavy gas in atmospheric nitrogen. Using two different methods to remove all known gases from air, Ramsay and Lord Rayleigh were able to announce in 1894 that they had found a monatomic, chemically inert gaseous element that constituted nearly 1 percent of the atmosphere; they named it argon.
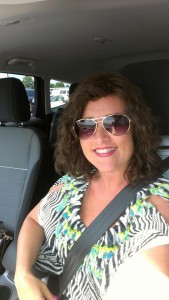
The following year, Ramsay liberated another inert gas from a mineral called cleveite. This proved to be helium, previously known only in the solar spectrum. In his book The Gases of the Atmosphere (1896), Ramsay showed that the positions of helium and argon in the periodic table of elements indicated that at least three more noble gases might exist. In 1898 Ramsay and the British chemist Morris W. Travers isolated these elements, called neon, krypton and xenon, from air brought to a liquid state at low temperature and high pressure.
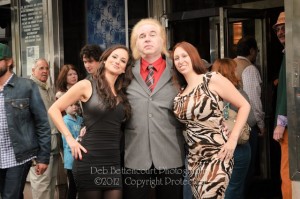
Sir William Ramsay worked with Frederick Soddy to demonstrate, in 1903, that alpha particles (helium nuclei) were continually produced during the radioactive decay of a sample of radium. Ramsay was awarded the 1904 Nobel Prize for Chemistry in recognition of “services in the discovery of the inert gaseous elements in air, and his determination of their place in the periodic system.”
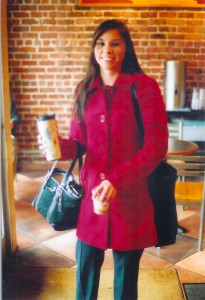
In 1897, J.J. Thomson discovered the electron using the cathode ray tube.
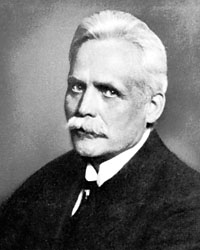
In 1898, Wilhelm Wien demonstrated that canal rays (streams of positive ions) can be deflected by magnetic fields, and that the amount of deflection is proportional to the mass-to-charge ratio. This discovery would lead to the analytical technique known as mass spectrometry.
Marie Sklodowska-Curie was a Polish-born French physicist and chemist who is famous for her pioneering research on radioactivity.
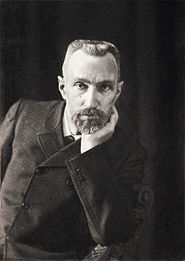
She and her husband Pierre are considered to have laid the cornerstone of the nuclear age with their research.
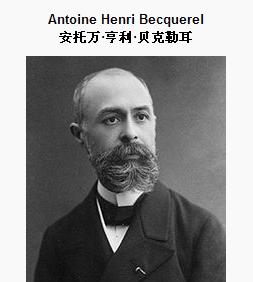
Marie was fascinated with the work of Henri Becquerel, a French physicist who discovered in 1896 that uranium casts off rays similar to the X-rays discovered by Wilhelm Röntgen.
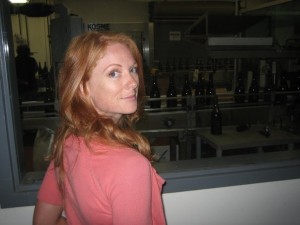
Marie Curie began studying uranium in late 1897 and theorized, according to a 1904 article she wrote for Century magazine, “that the emission of rays by the compounds of uranium is a property of the metal itself-that it is an atomic property of the element uranium independent of its chemical or physical state.”
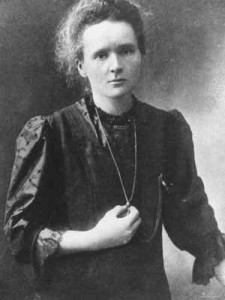
Curie took Becquerel’s work a few steps further, conducting her own experiments on uranium rays. She discovered that the rays remained constant, no matter the condition or form of the uranium. The rays, she theorized, came from the element’s atomic structure. This revolutionary idea created the field of atomic physics and the Curies coined the word radioactivity to describe the phenomena.
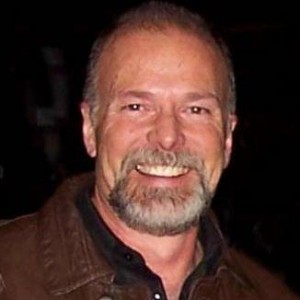
Pierre and Marie further explored radioactivity by working to separate the substances in uranium ores and then using the electrometer to make radiation measurements to ‘trace’ the minute amount of unknown radioactive element among the fractions that resulted. Working with the mineral pitchblende, the pair discovered a new radioactive element in 1898. They named the element polonium, after Marie’s native country of Poland.
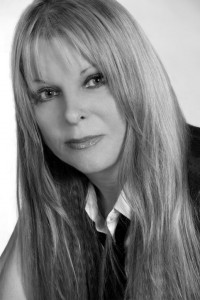
On December 21, 1898, the Curies detected the presence of another radioactive material in the pitchblende. They presented this finding to the Académie des Sciences on December 26, proposing that the new element be called radium.
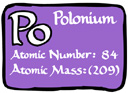
The Curies then went to work isolating polonium and radium from naturally occurring compounds to prove that they were new elements.
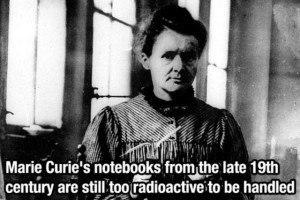
In 1902, the Curies announced that they had produced a decigram of pure radium, demonstrating its existence as a unique chemical element. While it took three years for them to isolate radium, they were never able to isolate polonium.
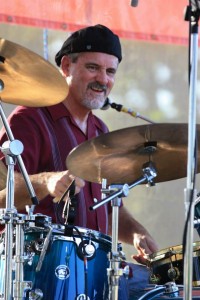
Along with the discovery of two new elements and finding techniques for isolating radioactive isotopes, Marie Curie oversaw the world’s first studies into the treatment of neoplasms using radioactive isotopes.
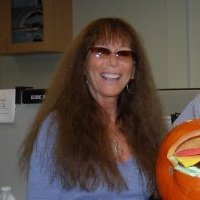
Marie Curie was awarded the 1903 Nobel Prize for Physics.
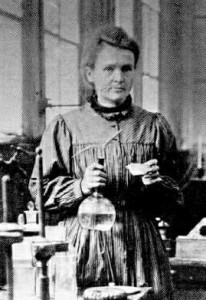
She was the sole winner of the 1911 Nobel Prize for Chemistry.

She was the first woman to win a Nobel Prize, and she is the only woman to win the award for work in two different fields.
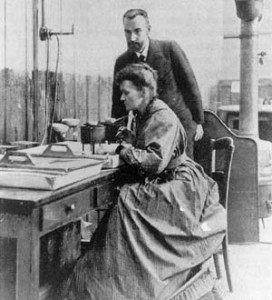
While working with Marie to extract pure substances from ores, an undertaking that really required industrial resources but that they achieved in relatively primitive conditions, Pierre himself concentrated on the physical study (including luminous and chemical effects) of the new radiations.
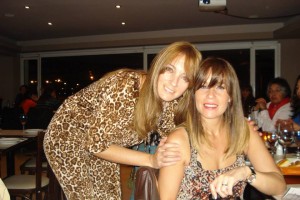
Through the action of magnetic fields on the rays given out by the radium, Pierre Curie proved the existence of particles electrically positive, negative, and neutral.
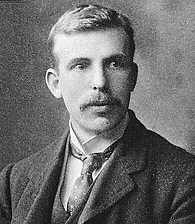
Ernest Rutherford would later call these particles alpha, beta, and gamma rays.
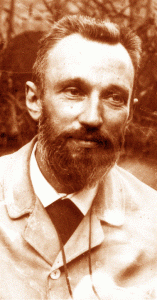
Pierre Curie then studied these radiations by calorimetry and also observed the physiological effects of radium, thus opening the way to radium therapy.
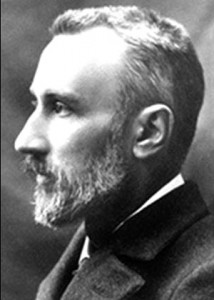
Among Pierre Curie’s discoveries were that ferromagnetic substances exhibited a critical temperature transition, above which the substances lost their ferromagnetic behavior – this is known as the “Curie point” He was elected to the Academy of Sciences (1905), having in 1903 jointly with Marie received the Royal Society’s prestigious Davy Medal and jointly with her and Becquerel the Nobel Prize for Physics. He was run over by a carriage in the rue Dauphine in Paris in 1906 and died instantly. His complete works were published in 1908.
New Zealand-born chemist and physicist Ernest Rutherford is considered to be “the father of nuclear physics.” Rutherford is best known for devising the names alpha, beta and gamma to classify various forms of radioactive “rays” which were poorly understood at his time (alpha and beta rays are particle beams, while gamma rays are a form of high-energy electromagnetic radiation).
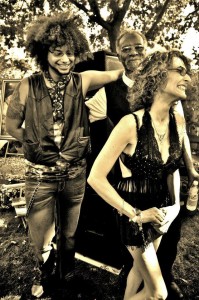
Rutherford deflected alpha rays with both electric and magnetic fields in 1903. Working with Frederick Soddy, Rutherford explained that radioactivity is due to the transmutation of elements, now known to involve nuclear reactions.
He also observed that the intensity of radioactivity of a radioactive element decreases over a unique and regular amount of time until a point of stability, and he named the halving time the “half-life”
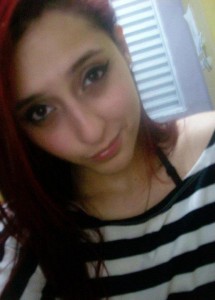
In 1901 and 1902 Rutherford worked with Frederick Soddy to prove that atoms of one radioactive element would spontaneously turn into another, by expelling a piece of the atom at high velocity.
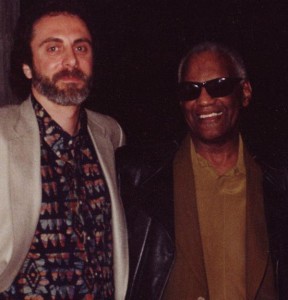
In 1906 at the University of Manchester, Rutherford oversaw an experiment conducted by his students Hans Geiger (known for the Geiger counter and Ernest Marsden. In the Geiger-Marsden experiment, a beam of alpha particles, generated by the radioactive decay of radon was directed normally onto a sheet of very thin gold foil in an evacuated chamber.
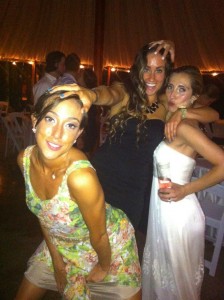
The alpha particles should all have passed through the foil and hit the detector screen, or have been deflected by, at most, a few degrees.
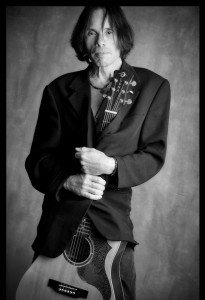
However, the actual results surprised Rutherford. Although many of the alpha particles did pass through as expected, many others were deflected at small angles while others were reflected back to the alpha source. Geiger, Marsden and Rutherford observed that a very small percentage of particles were deflected through angles much larger than 90 degrees. The gold foil experiment showed large deflections for a small fraction of incident particles.
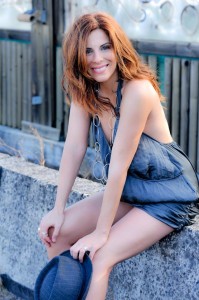
Rutherford realized that, because some of the alpha particles were deflected or reflected, the atom had a concentrated center of positive charge and of relatively large mass. Rutherford later termed this positive center the “atomic nucleus”.
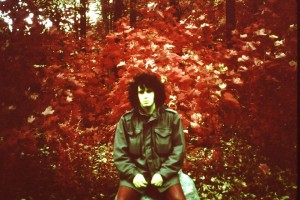
The alpha particles had either hit the positive center directly or passed by it close enough to be affected by its positive charge. Since many other particles passed through the gold foil, the positive centre would have to be a relatively small size compared to the rest of the atom – meaning that the atom is mostly open space.
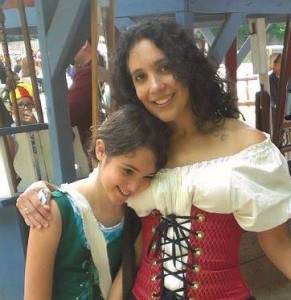
From these events and conclusions, Rutherford developed a model of the atom that was similar to the solar system, known as Rutherford model. Like planets, electrons orbited a central, sun-like nucleus. For his work with radiation and the atomic nucleus, Rutherford received the 1908 Nobel Prize in Chemistry.
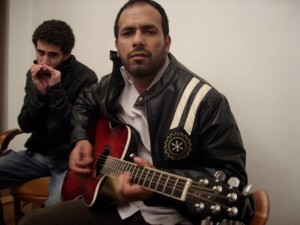
In 1903,Mikhail Tsvet invented chromatography, an important analytic technique.
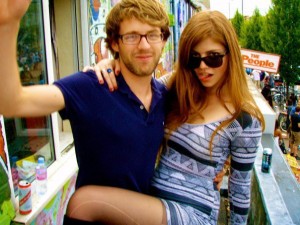
In 1904,Hantaro Nagaoka proposed an early nuclear model of the atom, where electrons orbit a dense massive nucleus.
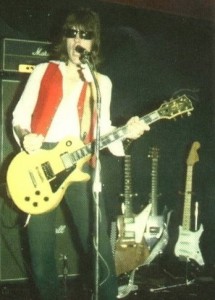
In 1905, Fritz Haber and Carl Bosch developed the Haber process for making ammonia, a milestone in industrial chemistry with deep consequences for agriculture. The Haber process, or Haber-Bosch process, combined nitrogen and hydrogen to form ammonia in industrial quantities for production of fertilizer and munitions. The food production for half the world’s current population depends on this method for producing fertilizer.
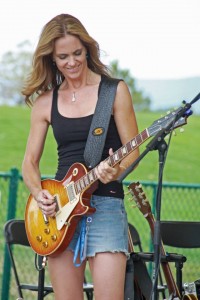
Haber, along with Max Born proposed the Born-Haber cycle as a method for evaluating the lattice energy of an ionic solid. Haber has also been described as the “father of chemical warfare” for his work developing and deploying chlorine and other poisonous gases during World War I.
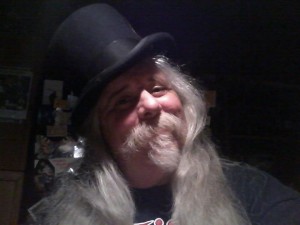
In the early twentieth century (1905), Albert Einstein explained Brownian motion in a way that definitively proved atomic theory.
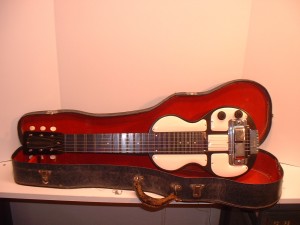
Leo Baekeland invnted bakelite one of the first commercially successful plastics.
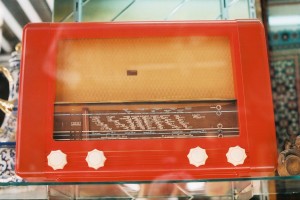
In 1909, American physicist Robert Andrews Millikan, who had studied in Europe under Walther Nernst and Max Planck, measured the charge of individual electrons with unprecedented accuracy through the oil drop experiment in which he measured the electric charges on tiny falling water (and later oil) droplets. His study established that any particular droplet’s electrical charge is a multiple of a definite, fundamental value, the electron’s charge, and thus a confirmation that all electrons have the same charge and mass.
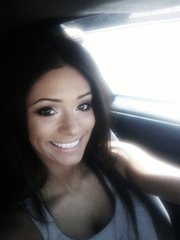
Beginning in 1912, Millikan spent several years investigating and finally proving Albert Einstein’s proposed linear relationship between energy and frequency, and providing the first direct photoelectric support for Planck’s constant. In 1923 Millikan was awarded the Nobel Prize for Physics.
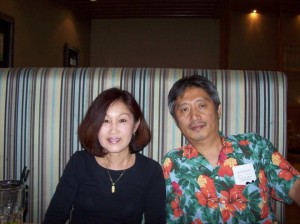
S.P.L. Sørensen invented the pH concept and developed methods for measuring acidity in 1909.
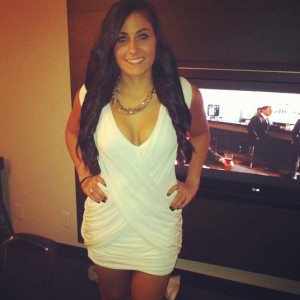
In 1911, Antonius Van den Broek proposed the idea that the elements on the periodic table are more properly organized by positive nuclear charge rather than atomic weight.
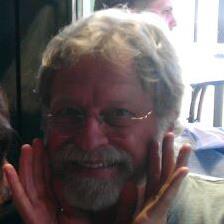
The first Solvay Conference (1911) was held in Brussels, bringing together most of the most prominent scientists of the day.
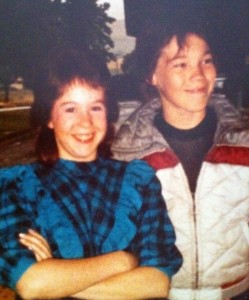
In 1912,William Henry Bragg and William Lawrence Bragg proposed Bragg’s law and established the field of X-ray crystallography, an important tool for elucidating the crystal structure of substances (1912).
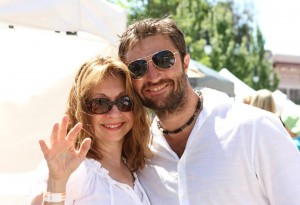
Also in 1912, Peter Debye developed the concept of molecular dipolarity to describe asymmetric charge distribution in some molecules.
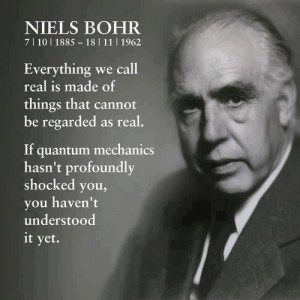
In 1913,Niels Bohr, a Danish physicist, introduced the concepts of quantum mechanics to atomic structure by proposing what is now known as the Bohr model of the atom, where electrons exist only in strictly defined circular orbits around the nucleus similar to rungs on a ladder.
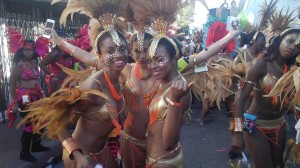
The Bohr Model is a planetary model in which the negatively-charged electrons orbit a small, positively-charged nucleus similar to the planets orbiting the sun (except that the orbits are not planar). The gravitational force of the solar system is mathematically akin to the attractive Coulomb (electrical) force between the positively-charged nucleus and the negatively-charged electrons.
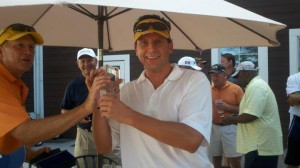
In the Bohr model, however, electrons orbit the nucleus in orbits that have a set size and energy. The energy levels are said to be quantized, which means that only certain orbits with certain radii are allowed. Orbits in between simply don’t exist.
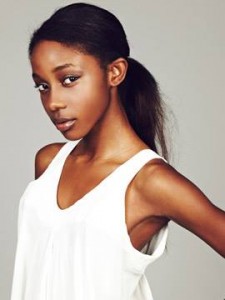
The energy of the orbit is related to its size – that is, the lowest energy is found in the smallest orbit.
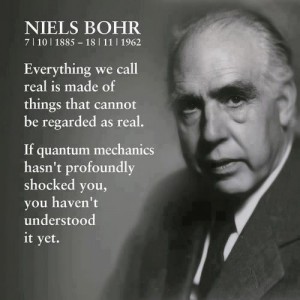
Bohr also postulated that electromagnetic radiation is absorbed or emitted when an electron moves from one orbit to another. Because only certain electron orbits are permitted, the emission of light accompanying a jump of an electron from an excited energy state to ground state produces a unique emission spectrum for each element.
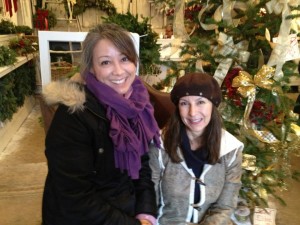
Neils Bohr also worked on the principle of complementarity which states that an electron can be interpreted in two mutually exclusive and valid ways. Electrons can be interpreted as wave or particle models. His hypothesis was that an incoming particle would strike the nucleus and create an excited compound nucleus. This formed the basis of his liquid drop model and later provided a theory base for the explanation of nuclear fission.
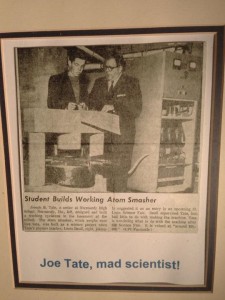
In 1913, Henry Mosely working from Van den Broek’s earlier idea, introduced the concept of atomic number to fix inadequacies in Mendeleev’s periodic table, which had been based on atomic weight.
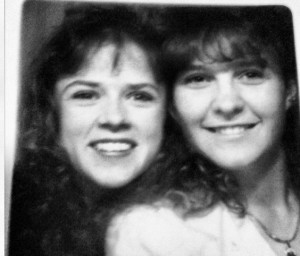
The peak of Frederick Soddy’s career in radiochemistry was in 1913 with his formulation of the concept of isotopes, which stated that certain elements exist in two or more forms which have different atomic weights but which are indistinguishable chemically. He is remembered for proving the existence of isotopes of certain radioactive elements, and is also credited, along with others, with the discovery of the element protactinium in 1917.
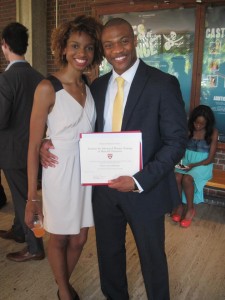
In 1913, J. J. Thomson expanded on the work of Wien by showing that charged subatomic particles can be separated by their mass-to-charge ratio, a technique known as mass spectrometry.
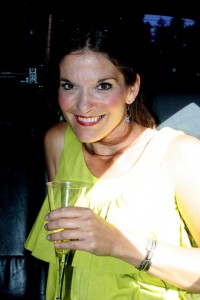
American physical chemist Gilbert N. Lewis laid the foundation of valence bond theory. He was instrumental in developing a bonding theory based on the number of electrons in the outermost “valence” shell of the atom. In 1902, while Lewis was trying to explain valence to his students, he depicted atoms as constructed of a concentric series of cubes with electrons at each corner. This “cubic atom” explained the eight groups in the periodic table and represented his idea that chemical bonds are formed by electron transference to give each atom a complete set of eight outer electrons (an “octet”).
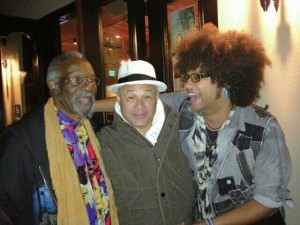
Lewis’s theory of chemical bonding continued to evolve and, in 1916, he published his seminal article “The Atom of the Molecule”, which suggested that a chemical bond is a pair of electrons shared by two atoms. Lewis’s model equated the classical chemical bond with the sharing of a pair of electrons between the two bonded atoms. Lewis introduced the “electron dot diagrams” in this paper to symbolize the electronic structures of atoms and molecules. Now known as Lewis structures they are discussed in virtually every introductory chemistry book.
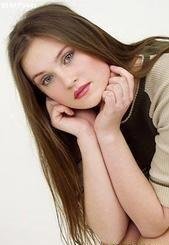
Shortly after publication of his 1916 paper, Lewis became involved with military research. He did not return to the subject of chemical bonding until 1923, when he masterfully summarized his model in a short monograph entitled Valence and the Structure of Atoms and Molecules.
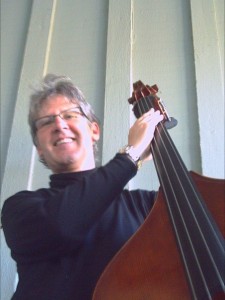
His renewal of interest in this subject was largely stimulated by the activities of the American chemist and General Electric researcher Irving Langmuir, who between 1919 and 1921 popularized and elaborated Lewis’s model. Langmuir subsequently introduced the term covalent bond.
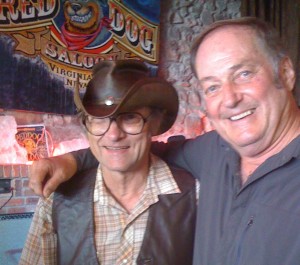
In 1921, Otto Stern and Walther Gerlach established the concept of quantum mechanical spin in subatomic particles.
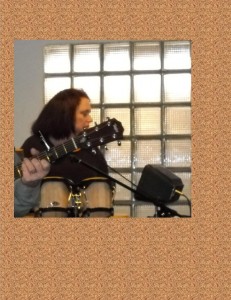
For cases where no sharing was involved, Lewis in 1923 developed the electron pair theory of acids and base.
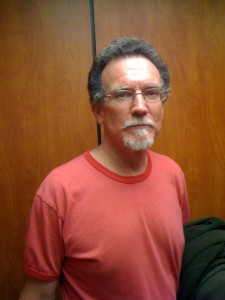
Lewis redefined an acid as any atom or molecule with an incomplete octet that was thus capable of accepting electrons from another atom. Bases were, of course, electron donors. His theory is known as the concept of Lewis acids and bases.
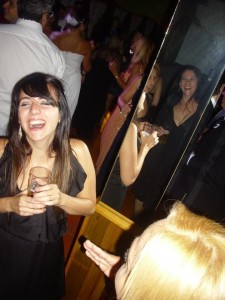
In 1923, G. N. Lewis and Merle Randall published Thermodynamics and the Free Energy of Chemical Substances, the first modern treatise on chemical thermodynamics.
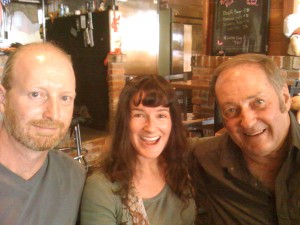
The 1920s saw a rapid adoption and application of Lewis’s model of the electron-pair bond in the fields of organic and coordination chemistry. In organic chemistry, this was primarily due to the efforts of the British chemists Arthur Lapworth, Robert Robinson, Thomas Lowry and Christopher Ingold.
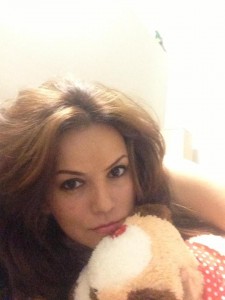
Lewis’s bonding model was promoted through the efforts of the American chemist Maurice Huggins and the British chemist Nevil Sidgwick.
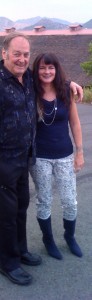
In 1924, French quantum physicist Louis de Broglie published his thesis, in which he introduced a revolutionary theory of electron waves based on wave-particle duality in his thesis. In his time, the wave and particle interpretations of light and matter were seen as being at odds with one another, but de Broglie suggested that these seemingly different characteristics were instead the same behavior observed from different perspectives, that particles can behave like waves, and waves (radiation) can behave like particles.
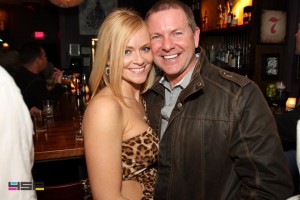
De Broglie’s proposal offered an explanation of the restriction motion of electrons within the atom. The first publications of de Broglie’s idea of “matter waves” had drawn little attention from other physicists, but a copy of his doctoral thesis chanced to reach Einstein, whose response was enthusiastic. Einstein stressed the importance of de Broglie’s work both explicitly and by building further on it.
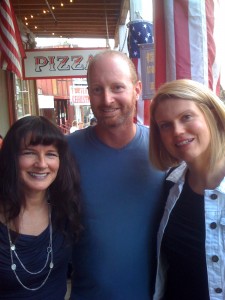
In 1925, Austrian-born physicist Wolfgang Pauli developed the Pauli exclusion principle, which states that no two electrons around a single nucleus in an atom can occupy the same quantum state simultaneously, as described by four quantum numbers.
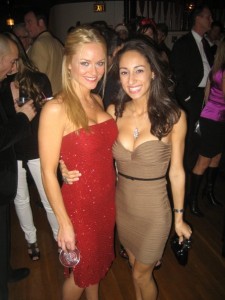
Pauli made major contributions to quantum mechanics and quantum field theory, and he was awarded the 1945 Nobel Prize for Physics for his discovery of the Pauli exclusion principle, as well as for solid-state physics, and he successfully hypothesized the existence of the neutrino.
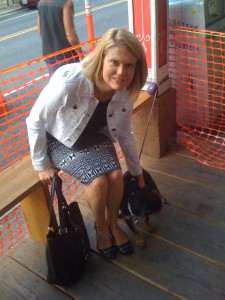
In addition to his original work, Wolfgang Pauli wrote masterful syntheses of several areas of physical theory that are considered classics of scientific literature.
In 1926 at the age of 39, Austrian theoretical physicist Erwin Schrödinger produced the papers that gave the foundations of quantum wave mechanics. In those papers he described his partial differential equation that is the basic equation of quantum mechanics and bears the same relation to the mechanics of the atom as Newton’s equations of motion bear to planetary astronomy.
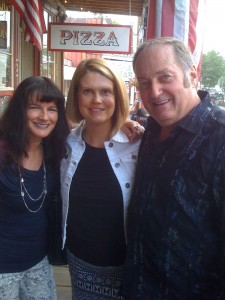
Schrödinger adopted a proposal made by Louis de Broglie in 1924 that particles of matter have a dual nature and in some situations act like waves, and he (Schrödinger) introduced a theory describing the behavior of such a system by a wave equation that is now known as the Schrödinger equation.
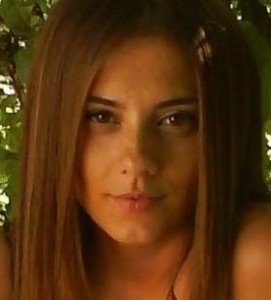
The solutions to Schrödinger’s equation, unlike the solutions to Newton’s equations, are wave functions that can only be related to the probable occurrence of physical events. The readily visualized sequence of events of the planetary orbits of Newton is, in quantum mechanics, replaced by the more abstract notion of probability. (This aspect of the quantum theory made Schrödinger and several other physicists profoundly unhappy, and he devoted much of his later life to formulating philosophical objections to the generally accepted interpretation of the theory that he had done so much to create.)
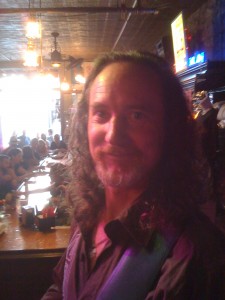
German theoretical physicist Werner Heisenberg was one of the key creators of quantum mechanics. In 1925, Heisenberg discovered a way to formulate quantum mechanics in terms of matrices. For that discovery, he was awarded the Nobel Prize for Physics for 1932.
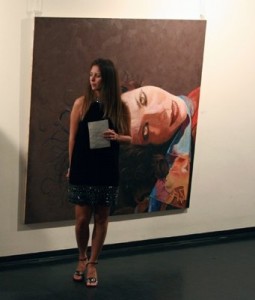
In 1927 Heisenberg published his uncertainty principle, upon which he built his philosophy and for which he is best known. Heisenberg was able to demonstrate that if you were studying an electron in an atom you could say where it was (the electron’s location) or where it was going (the electron’s velocity), but it was impossible to express both at the same time.
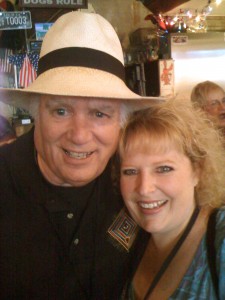
I think of Heisenberg’s principle this way. The very act of observing a sub atomic particle changes that particle. It is impossible to observe a sub atomic particle as it “really” is, because the observing of it changes it.
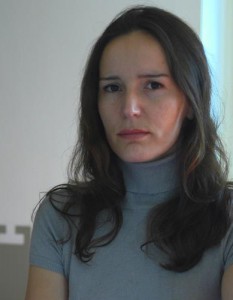
Heisenberg also made important contributions to the theories of the hydrodynamics of turbulenty flows, the atomic nucleus, ferromagnetism, cosmic rays and subatomic particles.
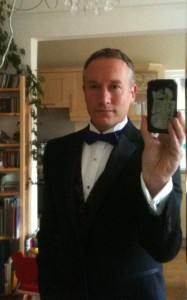
He was instrumental in planning the first West German nuclear reactor at Karlsruhe, together with a research reactor in München (Munich) in 1957.
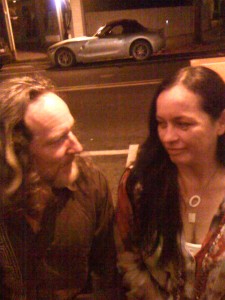
Considerable controversy surrounds Werner Heisenberg’s work on atomic research during World War II.
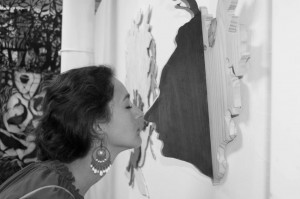
Some view the birth of quantum chemistry in the discovery of the Schrödinger equation and its application to the hydrogen atom in 1926. However, the 1927 article of Walter Heitler and Fritz Longon is often recognised as the first milestone in the history of quantum chemistry. This is the first application of quantum mechanics to the diatomic hydrogen molecule, and thus to the phenomenon of the chemical bond.
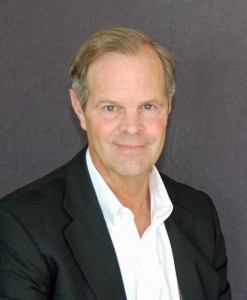
Werner von Braun was another figure of controversy for the same reason as was that other Werner… Heisenberg. Both men worked with people such as Edward Teller, Robert A. Millikan, Max Born, J. Robert Oppenheimer, Linus Pauling, Erich Hückel, Douglas Hartree and Vladimir Aleksandrovich Fock.
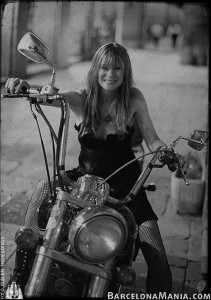
Skepticism remained as to the general power of quantum mechanics applied to complex chemical systems.
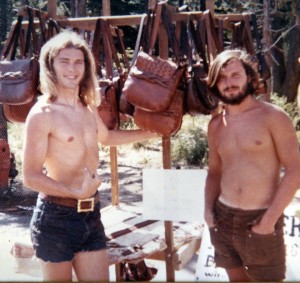
Hence the quantum mechanical methods developed in the 1930s and 1940s are often referred to as theoretical molecular or atomic physics to underline the fact that they were more the application of quantum mechanics to chemistry and spectroscopy than answers to chemically relevant questions.
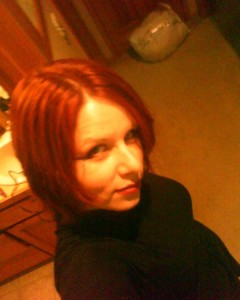
In the 1940s many physicists turned from molecular or atomic physics to nuclear physics (J. Robert Oppenheimer or Edward Teller).
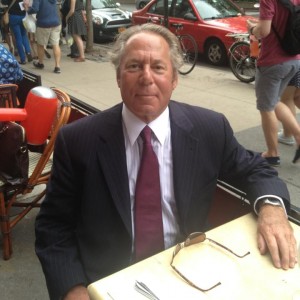
Clemens C.J. Roothaan wrote a seminal paper on Roothaan equations in 1951 that was a big step toward the solution of the self-consistent field equations for small molecules like hydrogen or nitrogen. Those computations were performed with the help of tables of integrals which were computed on the most advanced computers of the time.
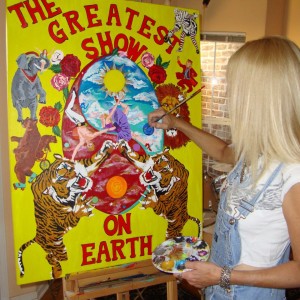
By the mid 20th century, in principle, the integration of physics and chemistry was extensive, with chemical properties explained as the result of the electronic structure of the atom. Linus Pauling’s book on The Nature of the Chemical Bond used the principles of quantum mechanics to deduce bond angles in ever-more complicated molecules.
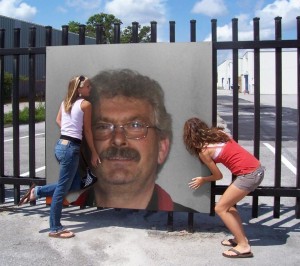
However, though some principles deduced from quantum mechanics were able to predict qualitatively some chemical features for biologically relevant molecules, they were, till the end of the 20th century, more a collection of rules, observations, and recipes than rigorous ab initio quantitative methods.
This heuristic approach triumphed in 1953 when James Watson and Francis Crick deduced the double helical structure of DNA by constructing models constrained by and informed by the knowledge of the chemistry of the constituent parts and the X-ray diffraction patterns obtained by Rosalind Franklin.
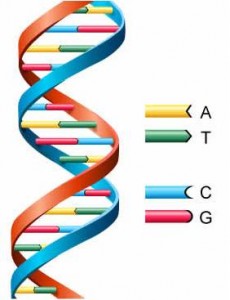
This discovery lead to an explosion of research into the biochemistry of life.
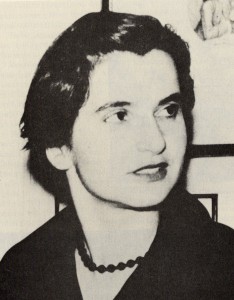
Rosalind Franklin was seriously taken advantage of in this research on DNA and her story is a sadly typical one. Added to the misogynistic tone of the proceedings, all too common in that era and that place, was a too familiar note of anti Semitism, common in the “upper” classes of that day.
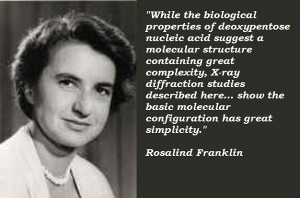
Rosalind Franklin’s DNA work achieved the most fame because DNA plays an essential role in cell metabolism and genetics, and the discovery of its structure helped her co-workers understand how genetic information is passed from parents to their offspring.
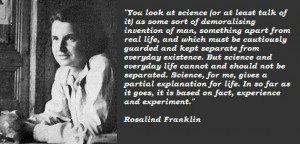
These co-workers, Watson and Crick, were more than a little unethical in their treatment of Rosalind Franklin. This is very disappointing in people of science.
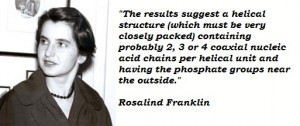
Her data were key to determining the structure for formulating Crick and Watson’s 1953 model of the structure of DNA.
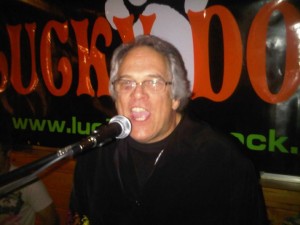
Also in 1953, the Miller-Urey experiment demonstrated that basic constituents of protein, simple amino acids, could themselves be built up from simpler molecules in a simulation of primordial processes on earth. Though many questions remain about the true nature of the origin of life, this was the first attempt by chemists to study hypothetical processes in the laboratory under controlled conditions.
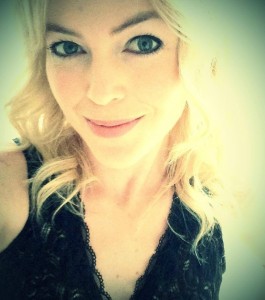
I remember being very excited when I heard of these experiments. I was at UC Berkeley in 1965 and a lot of that work was going on there. It seemed as if these scientists were creating the original earth’s atmosphere in a petri dish. I took LSD and thought about these experiments. It was all very dramatic and intensely interesting.
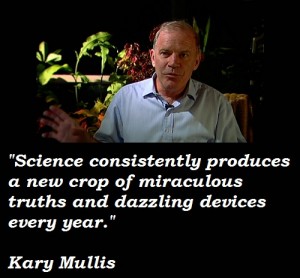
In 1983 Kary Mullis devised a method for the in-vitro amplification of DNA, known as polymerase chain reaction (PCR), which revolutionized the chemical processes used in the laboratory to manipulate it. PCR could be used to synthesize specific pieces of DNA using things similar to a PCR tube (some PCR tubes are manufactured here) and made possible the sequencing of the DNA of organisms, which culminated in the huge human genome project.

An important piece in the double helix puzzle was solved by one of Pauling’s students Matthew Meselson and Frank Stahl, and the result of their collaboration (the Meselson-Stahl experiment has been called as “the most beautiful experiment in biology”.
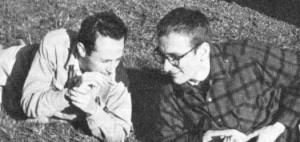
They used a centrifugation technique that sorted molecules according to differences in weight. Because nitrogen atoms are a component of DNA, they were labelled and therefore tracked in replication in bacteria.
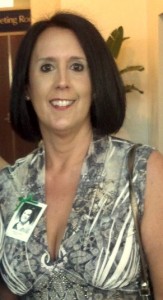
In 1970, John Pople developed the Gaussian program which simplified computational chemistry calculations.
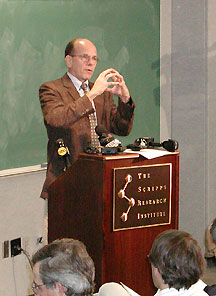
Yves Chauvin offered an explanation of the reaction mechanism ofolefin metathesis reactions in 1973 and in 1975, Karl Barry Sharpless and his group discovered stereoselective oxidation reactions including the Sharpless epoxidation, Sharpless asymmetric dihydroxylation and the Sharpless oxyamination.
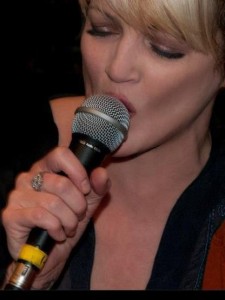
In 1985, Harold Kroto, Robert Curl and Richard Smalley discovered fullerenes.
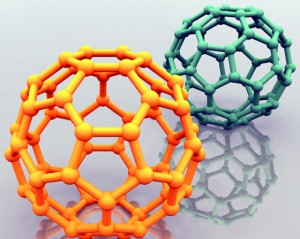
Fullerenes are a class of large carbon molecules superficially resembling the geodesic dome designed by architect R. Buckminster Fuller.
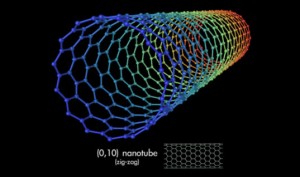
Sumio Iijima used electron microscopy in 1991 to discover a type of cylindrical fullerene known as a carbon nanotube though earlier work had been done in the field as early as 1951.
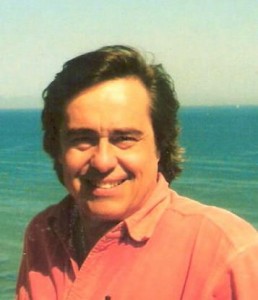
This material is an important component in the field of nanotechnology.
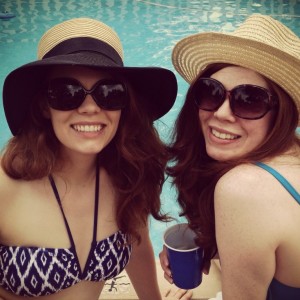
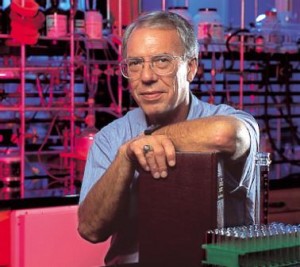
In 1994, Robert A. Holton and his group achieved the first total synthesis of Taxol.
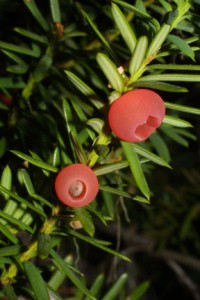
Isolated from the bark of the relatively rare and slow-growing pacific yew tree over twenty years ago, taxol is the most promising new antitumor agent for the treatment of ovarian and breast cancers.
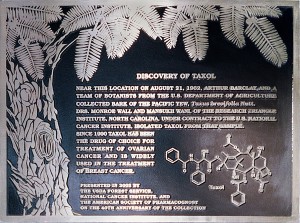
Taxol has a unique mechanism of action, blocking cell division by binding and stabilizing microtubules, structures which comprise the cytoskeleton and the mitotic spindle.
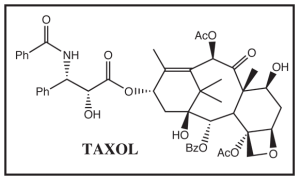
A few years ago, Holton’s group developed an efficient semisynthesis of taxol which will provide the commercial supply, and this has made it unnecessary to destroy the environment through the harvest of yew trees.
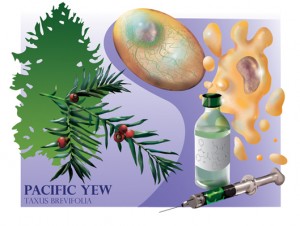
The yew tree has long been recognized as a tree of strong medicine. Just today I read an account in Julius Caesar of the yew tree’s powers.
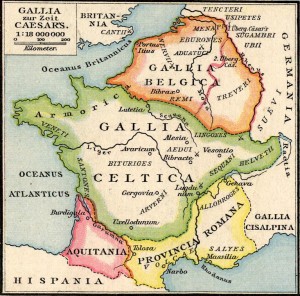
Catuvoleus, rex dimidiae partis Eburonum qui inierat consilium una cum Ambiorige, jam confectus aetate, quum posset non ferre laborem aut belli aut fugae, detestatus Ambiorigem omnibus precibus qui fuisset auctor ejus consilii, exanimavit se taxo (cujus est magna copia in Gallia que Germania). Liber VI De Bello Gallico
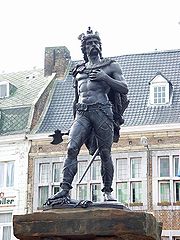
Catuvoleus, king of half of the Eburones, who had entered into counsel with Ambiorix, now worn out with age, since he could not bear the fatigue of either war or flight, cursed Ambiorix with all kinds of imprecations since he had been the author of this plan, and then killed himself by eating yew leaves (the yew grows in great abundance in Gaul and Germany). Book VI The Gallic War
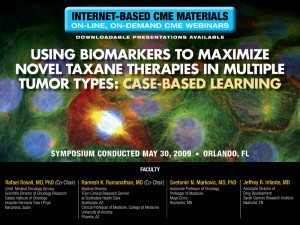
All species of yew contain highly poisonous (and, paradoxically, highly beneficial) alkaloids known as taxanes, with some variation in the exact formula of the alkaloid between the species. All parts of the tree except the arils contain the alkaloid. The arils are edible and sweet, but the seed is dangerously poisonous. Unlike birds’ stomachs, the human stomach can break down the seed coat and release the taxanes into the body.
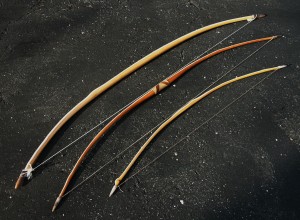
The yew is an amazing tree with a long history. The man found in the ice in Italy who died five thousand years ago, Ötzi, as he is called, carried a bow made of yew. Yew is also associated with Wales and England because of the longbow, an early weapon of war developed in northern Europe, and as the English longbow which was famously used at the battle of Agincourt.
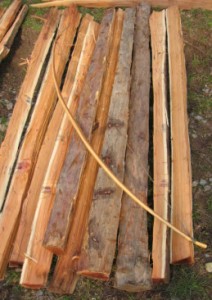
Yew is the wood of choice for longbow making; the bows are constructed so that the heartwood of yew is on the inside of the bow while the sapwood is on the outside. This takes advantage of the natural properties of yew wood since the heartwood resists compression while the sapwood resists stretching.
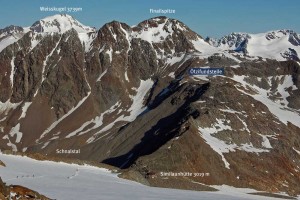
The word yew is from Proto-Germanic. Baccata is Latin for bearing red berries. The word yew as it was originally used seems to refer to the color brown.
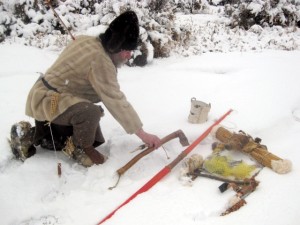
The yew (?????) was known to Theophrastus who noted its preference for mountain coolness and shade, its evergreen character and its slow growth.
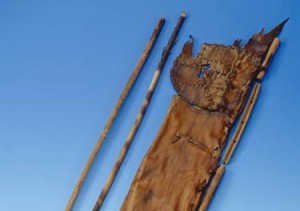
Most romance languages kept a version of the Latin word taxus (Italian tasso, Corsican tassu, Occitan teis, Catalan teix, Gasconic tech, Spanish tejo, Portuguese teixo, Galician teixo and Romanian tis?) from the same root as toxic.

In Slavic languages, the same root (presumably borrowed from Romanian) is preserved: Russian tiss (???), Slovenian tisa, Serbiantisa (????). In Albanian it is named tis.
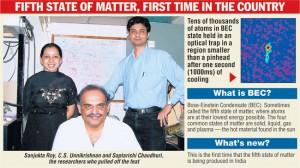
In 1995,Eric Cornell and Carl Wieman produced the first Bose-Einstein condensate, a substance that displays quantum mechanical properties on the macroscopic scale.
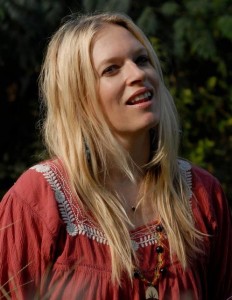
Before the 20th century, chemistry was defined as the science of the nature of matter and its transformations. It was therefore clearly distinct from physics which was not concerned with such dramatic transformation of matter.

Moreover, in contrast to physics, chemistry was not using much of mathematics. Some scientists, such as Auguste Comte were particularly reluctant to use mathematics within chemistry.
Every attempt to employ mathematical methods in the study of chemical questions must be considered profoundly irrational and contrary to the spirit of chemistry…. if mathematical analysis should ever hold a prominent place in chemistry – an aberration which is happily almost impossible – it would occasion a rapid and widespread degeneration of that science.
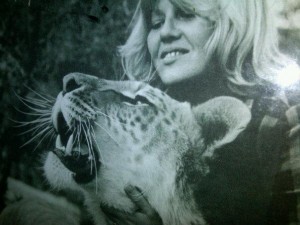
However, in the second part of the 19th century, the situation changed and August Kekulé wrote in 1867:
I rather expect that we shall someday find a mathematico-mechanical explanation for what we now call atoms which will render an account of their properties.
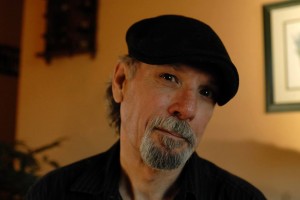
After the discovery by Rutherford and Bohr of the atomic structure in 1912, and by Marie and Pierre Curie of radioactivity, scientists had to change their viewpoint on the nature of matter.
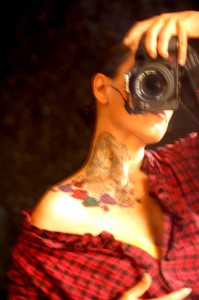
The experience acquired by chemists was no longer pertinent to the study of the whole nature of matter but only to aspects related to the electron cloud surrounding the atomic nuclei and the movement of the latter in the electric field induced by the former.
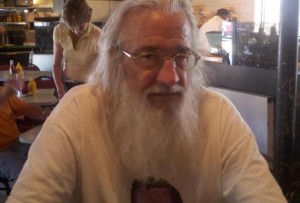
The range of chemistry was thus restricted to the nature of matter around us in conditions which are not too far (or exceptionally far) from standard conditions for temperature and pressure and in cases where the exposure to radiation is not too different from the natural microwave, visible or UV radiations on Earth. Chemistry was therefore re-defined as the science of matter that deals with the composition, structure, and properties of substances and with the transformations that they undergo.
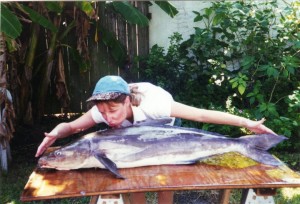
However the meaning of matter used here relates explicitly to substances made of atoms and molecules, disregarding the matter within the atomic nuclei and its nuclear reaction or matter within highly ionized plasmas.
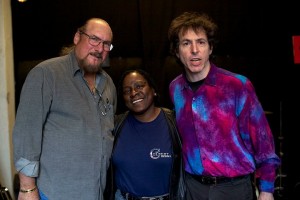
This does not mean that chemistry is never involved with plasma or nuclear sciences or even bosonic fields nowadays.
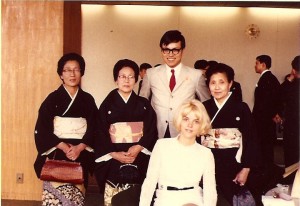
Areas such as Quantum Chemistry and Nuclear Chemistry are currently well developed and formally recognized sub-fields of study under the Chemical sciences (Chemistry).
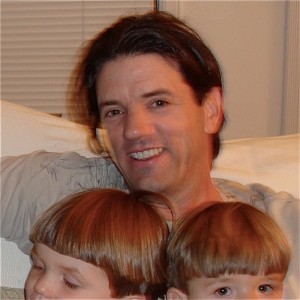
What is now formally recognized, however, as subject of study under the Chemistry category as a science is always based on the use of concepts that describe or explain phenomena either from matter or to matter in the atomic or molecular scale.
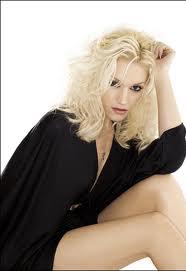
This includes the study of the behavior of many molecules as an aggregate or the study of the effects of a single proton on a single atom.
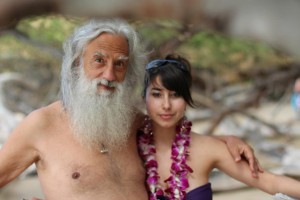
Physicists and not chemists deal with different (more “exotic”) types of matter (e.g. Bose-Einstein condensate, Higgs Boson, dark matter, naked singularity).
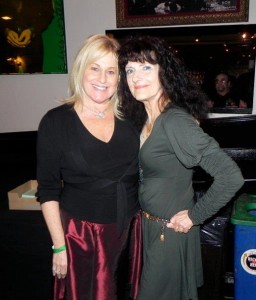
The field of chemistry is still, on our human scale, very broad and the claim that chemistry is everywhere is, of course, accurate.
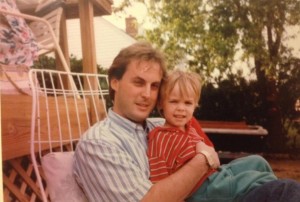
The later part of the nineteenth century saw a huge increase in the exploitation of petroleum extracted from the earth for the production of a host of chemicals, which largely replaced the use of whale oil, coal tar and naval stores.
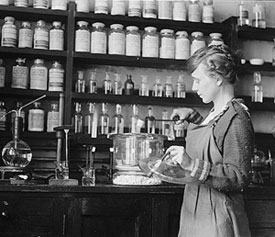
Large scale production and refinement of petroleum provided feedstocks for liquid fuels such asgasoline and diesel, solvents, lubricants, asphalt and waxes.
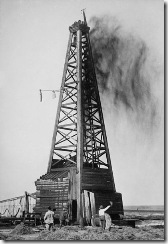
Refined petroleum is also the fundamental ingredient in many of the common materials of the modern world.
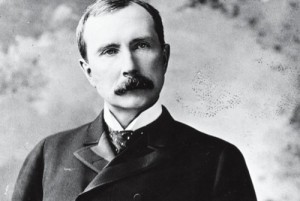
Materials such as synthetic fibers, plastics, paints, detergents, pharmaceuticals, adhesives and for ammonia as fertilizer.
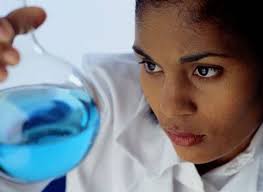
Many of these required new catalysts to be used practically and this naturally involved chemistry.
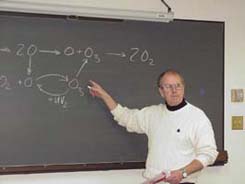
In the mid-twentieth century, control of the electronic structure of semiconductor materials was made precise by the creation of large ingots of extremely pure single crystals of silicon and geranium.
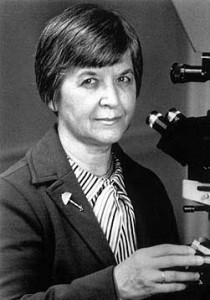
Accurate control of their chemical composition by doping with other elements made the production of the solid state transistor in 1951.
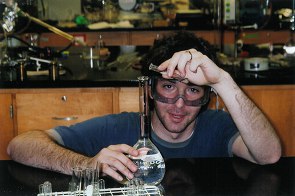
Chemistry also made possible the production of the tiny integrated circuits in the machine that I am using to write this.
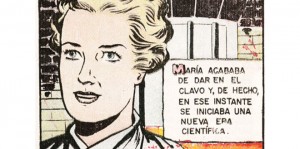
So, here is a salute to all the women and men who worked through all the ages to further the cause of chemistry.
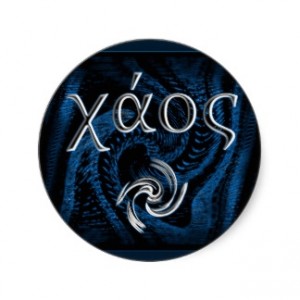
See you next week?
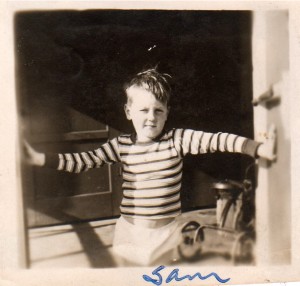
Sam Andrew
_____________________________________